Cerebral circulation
Cerebral circulation is the movement of blood through the network of cerebral arteries and veins supplying the brain that is essential for survival. The rate of the cerebral blood flow in the adult is typically 750 milliliters per minute, representing 15% of the cardiac output. The arteries deliver oxygenated blood, glucose and other nutrients to the brain, and the veins carry deoxygenated blood back to the heart, removing carbon dioxide, lactic acid, and other metabolic products. Regulation of cerebral blood flow is vital because it allows the brain to get its nutrients and oxygen even when the blood pressure in the body changes.
Since the brain is very vulnerable to compromises in its blood supply, the cerebral circulatory system has many safeguards including autoregulation of the blood vessels and the failure of these safeguards can result in a stroke. The amount of blood that the cerebral circulation carries is known as cerebral blood flow. Sudden intense accelerations change the gravitational forces perceived by bodies and can severely impair cerebral circulation and normal functions to the point of becoming serious life threatening conditions.
Although the brain is only 2% of the adult body weight, it receives 15% of the blood (about 750 mL/min.) and consumes 20% of its oxygen and glucose, even when you are resting. Because neurons have such a high demand for ATP (adenosine triphosphate is a high-energy molecule found in every cell, its job is to store and supply the cell with needed energy) and therefore glucose and oxygen, the constancy of blood supply is especially critical to the nervous system. Neurons synthesize ATP almost exclusively from glucose via reactions that use oxygen. When the activity of neurons and neuroglia increases in a particular region of the brain, blood flow to that area also increases. A mere 10-second interruption in blood flow can cause loss of consciousness, such as when you stand up too quickly after sitting for a long period of time. Typically, an interruption in blood flow for 1 or 2 minutes can significantly impair neural function; and total deprivation of oxygen for about 4 minutes usually causes irreversible brain damage. Because virtually no glucose is stored in the brain, the supply of glucose also must be continuous. If blood entering the brain has a low level of glucose, mental confusion, dizziness, convulsions, and loss of consciousness may occur. People with diabetes must be vigilant about their blood sugar levels because these levels can drop quickly, leading to diabetic shock, which is characterized by seizure, coma, and possibly death.
The brain receives its arterial supply from two pairs of vessels, the vertebral and internal carotid arteries, which are interconnected in the cranial cavity to produce a cerebral arterial circle (of Willis). The two vertebral arteries enter the cranial cavity through the foramen magnum and just inferior to the pons fuse to form the basilar artery. The two internal carotid arteries enter the cranial cavity through the carotid canals on either side.
The dural venous sinuses drain into the internal jugular veins to return blood from the head to the heart.
Despite blood’s critical importance to the brain, blood is also a source of antibodies, macrophages, bacterial toxins, and other potentially harmful agents. Damaged brain tissue is essentially irreplaceable, and the brain therefore must be well protected. Consequently, there is a brain barrier system that strictly regulates what can get from the bloodstream into the tissue fluid of the brain.
There are two potential points of entry that must be guarded:
- Blood capillaries throughout the brain tissue and
- Capillaries of the choroid plexuses.
At the blood capillaries that are present throughout the brain tissue, the brain is well protected by the blood–brain barrier (BBB). The blood–brain barrier (BBB) consists mainly of tight junctions that seal together the endothelial cells of brain blood capillaries and a thick basement membrane that surrounds the capillaries. Astrocytes are one type of neuroglia; the processes of many astrocytes press up against the capillaries and secrete chemicals that maintain the “tightness” of the tight junctions. The blood–brain barrier (BBB) allows certain substances in blood to enter brain tissue and prevents passage to others. Lipid-soluble substances (including oxygen [O2], carbon dioxide [CO2]), steroid hormones, alcohol, barbiturates, nicotine, and caffeine) and water molecules easily cross the blood–brain barrier by diffusing across the lipid bilayer of endothelial cell plasma membranes. A few water-soluble substances, such as glucose, quickly cross the BBB by facilitated transport. Other water-soluble substances, such as most ions, are transported across the BBB very slowly. Still other substances— proteins and most antibiotic drugs—do not pass at all from the blood into brain tissue. Trauma, certain toxins, and inflammation can cause a breakdown of the BBB.
Figure 1. Brain blood supply
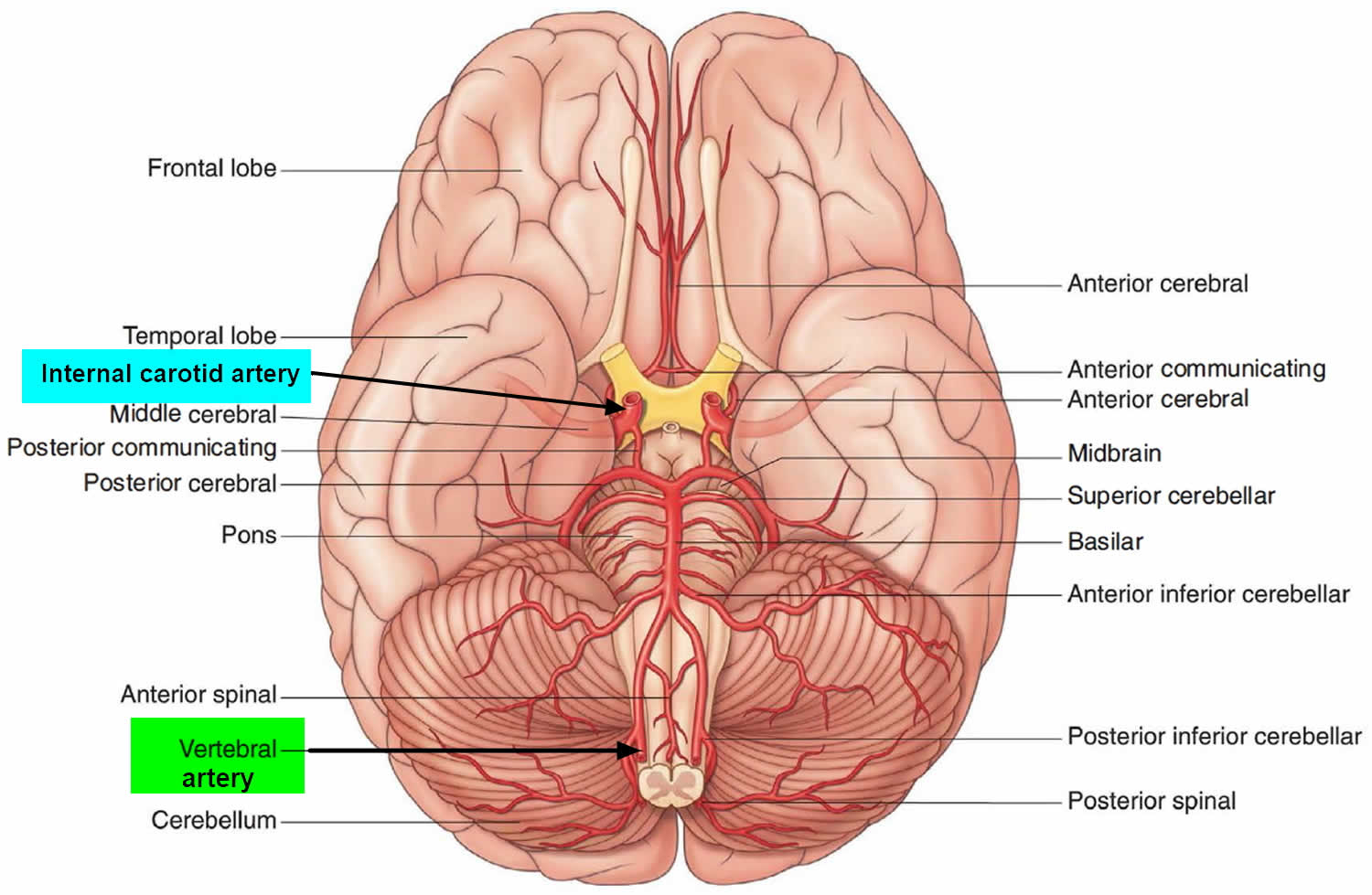
Cerebral blood flow
Cerebral blood flow is the blood supply to the brain in a given period of time. In an adult, cerebral blood flow is typically 750 milliliters per minute or 15% of the cardiac output. Cerebral blood flow is tightly regulated to meet the brain’s metabolic demands. Too much blood (a condition known as hyperemia) can raise intracranial pressure (ICP), which can compress and damage delicate brain tissue. Too little blood flow (ischemia) results if blood flow to the brain is below 18 to 20 ml per 100 g per minute, and tissue death occurs if flow dips below 8 to 10 ml per 100 g per minute. In brain tissue, a biochemical cascade known as the ischemic cascade is triggered when the tissue becomes ischemic, potentially resulting in damage to and the death of brain cells. Medical professionals must take steps to maintain proper cerebral blood flow in patients who have conditions like shock, stroke, cerebral edema, and traumatic brain injury.
Cerebral blood flow is determined by a number of factors, such as viscosity of blood, how dilated blood vessels are, and the net pressure of the flow of blood into the brain, known as cerebral perfusion pressure, which is determined by the body’s blood pressure. Cerebral perfusion pressure (CPP) is defined as the mean arterial pressure (MAP) minus the intracranial pressure (ICP). In normal individuals it should be above 50 mm Hg. Intracranial pressure should not be above 15 mm Hg (ICP of 20 mm Hg is considered as Intracranial Hypertension) 1. Cerebral blood vessels are able to change the flow of blood through them by altering their diameters in a process called autoregulation; they constrict when systemic blood pressure is raised and dilate when it is lowered 2. Arterioles also constrict and dilate in response to different chemical concentrations. For example, they dilate in response to higher levels of carbon dioxide in the blood and constrict to lower levels of carbon dioxide 2.
For example, assuming a person with an arterial partial pressure of carbon dioxide (PaCO2) of 40 mmHg (normal range of 38 – 42 mmHg) 3 and a cerebral blood flow of 50 ml per 100g per min. If the PaCO2 dips to 30 mmHg, this represents a 10 mmHg decrease from the initial value of PaCO2. Consequently, the cerebral blood flow decreases by 1ml per 100g per min for each 1mmHg decrease in PaCO2, resulting in a new cerebral blood flow of 40ml per 100g of brain tissue per minute. In fact, for each 1 mmHg increase or decrease in PaCO2, between the range of 20–60 mmHg, there is a corresponding cerebral blood flow change in the same direction of approximately 1–2 ml/100g/min, or 2–5% of the cerebral blood flow value 4. This is why small alterations in respiration pattern can cause significant changes in global cerebral blood flow, specially through PaCO2 variations 4.
Cerebral blood flow (CBF) is equal to the cerebral perfusion pressure (CPP) divided by the cerebrovascular resistance (CVR) 5:
- CBF = CPP / CVR
Cerebral perfusion pressure (CPP) is defined as the difference between mean arterial pressure (MAP) and intracranial pressure (ICP) or central venous pressure (CVP), whichever is the highest. Mean arterial pressure (MAP) is the diastolic pressure plus one third of the pulse pressure (difference between the systolic and diastolic).
- CPP = MAP – ICP (or CVP, whichever is the highest)
A CPP less than 70 mmHg can lead to a rapid decrease in jugular venous bulb saturation (normal range 65%-75%) because of increased oxygen extraction
A number of studies on patients with severe head injuries have shown an increase in mortality and poor outcome when CPP falls to less than 70 mmHg for a sustained period.
Control of cerebral blood flow (CBF) is considered in terms of the factors affecting cerebral perfusion pressure (CPP) and the factors affecting cerebrovascular resistance (CVR). Cerebrovascular resistance (CVR) is controlled by four major mechanisms:
- Metabolic control (or ‘metabolic autoregulation’)
- Pressure autoregulation
- Chemical control (by arterial pCO2 and pO2)
- Neural control
Cerebral circulation anatomy
Blood supply to the brain is normally divided into anterior and posterior segments, relating to the different arteries that supply the brain. The two main pairs of arteries are the Internal carotid arteries (supply the anterior brain) and vertebral arteries (supplying the brainstem and posterior brain).
Classically the internal carotid arteries on both sides are referred to as the anterior circulation, while the vertebral-basilar arterial system composes the posterior circulation 6. At the central cranial base, the anterior and posterior circulation connect to form an anastomotic ring called “Circle of Willis”, which provides backup circulation to the brain. The sides of the Circle of Willis are made of the anterior cerebral arteries, the posterior cerebral arteries, anterior communicant branch which bridge both anterior cerebral arteries and the posterior communicant arteries which links internal carotid artery and posterior cerebral arteries on each hemibrain 7.
Each internal carotid artery divides into 3 terminal branches:
- Anterior cerebral arteries (ACA),
- Middle cerebral artery (MCA),
- Anterior choroidal artery.
The posterior cerebral arteries are the terminal branches of the vertebrobasilar artery.
Anatomists describe different segments of anterior cerebral arteries, middle cerebral artery, and posterior cerebral arteries based on the bifurcating pattern 8. Hence, anterior cerebral arteries is subdivided in 9:
- A1: Horizontal or pre-communicating begins at the carotid bifurcation and ends at the level of anterior communicant branch.
- A2: Vertical or post communicating segment or pre callosal begins at anterior communicant branch and ends at the junction rostrum-genu of the corpus callosum.
- A3: Pre-callosal
- A4: Supra-callosal
- A5: Postero-callosal
A3 together with A4 and A5 are referred to as peri-callosal artery.
Middle cerebral artery is subdivided in 9:
- M1: Sphenoidal
- M2: Insular
- M3: Opercular
- M4: Cortical
Posterior cerebral artery is subdivided in 9:
- P1: Pre-communicating
- P2: Post-communicating
- P3: Quadrigeminal
- P4: Calcarine
Anterior cerebral arteries, middle cerebral artery, and posterior cerebral arteries harbor important perforating branches which supply vital structures such as the pituitary gland and its infundibular stalk, the optic chiasm, hypothalamus and thalamus, midbrain and basal ganglia.
Following are the main cerebral arteries, their perforating branches, and related supplied territory.
Anterior cerebral circulation
The anterior cerebral circulation is the blood supply to the anterior portion of the brain including eyes. It is supplied by the following arteries:
- Internal carotid arteries (ICA): These large arteries are the medial branches of the common carotid arteries which enter the skull, as opposed to the external carotid branches which supply the facial tissues; the internal carotid artery branches into the anterior cerebral artery and continues to form the middle cerebral artery.
- Anterior cerebral artery (ACA)
- Anterior communicating artery: Connects both anterior cerebral arteries, within and along the floor of the cerebral vault.
- Middle cerebral artery (MCA)
Anterior cerebral artery, anterior choroidal artery and its branches
Recurrent artery (of Heubner) is the largest artery arising from A1 or proximal A2 10. It passes above and laterally to the optic chiasm and penetrates the anterior perforated substance. The recurrent artery supplies the head of the caudate nucleus, anterior third of the putamen, anterior part of the globus pallidus externus, anteroinferior portion of the anterior limb of the internal capsule, and the uncinate fasciculus, and, rarely, the anterior hypothalamus.
Basal perforating arteries (medial lenticulostriate arteries) arising from anterior cerebral artery and anterior communicating artery supply the anterior perforated substance, the dorsal surface of the optic nerve and optic chiasm, the optic tract, the suprachiasmatic portion of the hypothalamus, the rostrum of the corpus callosum, the lower surface of the frontal lobe and the medial part of the Sylvian fissure 11.
Peri-callosal artery is the distal part of the artery surrounding the corpus callosum. It gives rise to its largest branch: the callosomarginal artery. The callosomarginal artery is recognizable as it courses in or near the cingulate sulcus. Both callosomarginal and distal peri-callosal artery give 5 main cortical branches. They are orbitofrontal, frontopolar, internal frontal, paracentral and parietal arteries. The cortical branches supply the basal surface of the frontal lobe, the superior frontal gyrus and the anterior two-thirds of the medial hemisphere (including the pre-central, central and post-central gyri). Some distal ACA may supply part of the contralateral hemisphere.
Anterior choroidal artery
The anterior choroidal artery is a small but relatively constant vessel that arises from the posteromedial aspect of the supra-clinoid internal carotid artery a short distance above the posterior communicant arteries origin. The anterior choroidal artery may exist either as a single trunk or a plexus of small vessels. Anterior choroidal artery territory is variable and may include the optic tract, the posterior limb of the internal capsule, cerebral peduncle, choroid plexus, and medial temporal lobe 12.
Middle cerebral artery
The proximal segment (M1) courses laterally to the optic chiasm to reach the medial entrance of the Sylvian fissure. During its journey, it describes two parts: pre and post bifurcation. This main trunk mainly bifurcates but, in some instances, may trifurcate. M1 harbors perforating branches, named lateral lenticulo-striate arteries, which supply most of the caudate nucleus, internal capsule, basal ganglia. Its cortical branches supply the anterior pole of the temporal lobe. The insular segment (M2) consists of 6 to 8 main arteries that lie over the insula and end on top of the circular sulcus. The distal segments M3 and M4 lie from the surface of the lateral cerebral fissure to most of the lateral surface of the brain. Its cortical branches are orbitofrontal, pre-frontal, pre and postcentral sulcus, parietal, angular, temporal, and temporal-occipital arteries. M3 and M4 territory cover almost the entire lateral surface of the brain. Although anastomoses are developed between the anterior cerebral artery, middle cerebral artery and posterior cerebral artery arterial territories, watershed areas exist on the cortical surface leading to vulnerable brain areas during hypotensive events.
Posterior cerebral circulation
The posterior cerebral circulation is the blood supply to the posterior portion of the brain, including the occipital lobes, cerebellum and brainstem. It is supplied by the following arteries:
- Vertebral arteries: These smaller arteries branch from the subclavian arteries which primarily supply the shoulders, lateral chest and arms. Within the cranium the two vertebral arteries fuse into the basilar artery.
- Posterior inferior cerebellar artery (PICA)
- Basilar artery: Supplies the midbrain, cerebellum, and usually branches into the posterior cerebral artery
- Anterior inferior cerebellar artery (AICA)
- Pontine branches
- Superior cerebellar artery (SCA)
- Posterior cerebral artery (PCA)
- Posterior communicating artery
Posterior cerebral artery
From the basilar bifurcation to the junction with Pcom A, the proximal segment (P1) of posterior cerebral artery gives numbers of important perforating branches that supply the brainstem, thalamus, oculomotor and trochlear nuclei. P2 courses between the junction and the posterior aspect of the midbrain where it gives thalamoperforating, thalamogeniculate, peduncular perforating, posterior choroidal and posterior temporal arteries. The distal part of posterior cerebral artery (P3 and P4) extends from the quadrigeminal plate to the calcarine fissure where they supply the occipital lobe, part of the parietal and temporal lobe and the posterior third of the medial brain hemisphere.
Vertebrobasilar system
The intradural portion of the vertebral artery courses anteromedially through the foramen magnum, runs superomedially toward the midline where the two vertebral arteries unite to form the basilar artery 13. During its journey to basilar artery, vertebral artery gives the posteroinferior cerebellar artery (PICA), the anterior spinal artery which supplies the upper spinal cord, lateral medulla, tonsils, the vermis, and the inferior cerebellar hemisphere 14.
From the confluence of the vertebral arteries, the Basilar courses superiorly in front of the medulla, pons and bifurcate at the junction pons/mesencephalon where it gives the posterior cerebral arteries. Along its course, basilar artery gives the anterior inferior cerebellar arteries (AICA), pontine perforating branches and the superior cerebellar arteries (SCA). Basilar artery supplies the brainstem, midbrain, part of the thalamus, posterior internal capsule, the mid- and upper cerebellum and vermis.
Venous circulation of the brain
The cerebral venous system is a freely communicating and interconnected system comprised of dural sinuses and cerebral veins 15. Venous outflow from the cerebral hemispheres consists of two groups of valveless veins, which allow for drainage: the superficial cortical veins and the deep or central veins (Figure 2).
The superficial cortical veins are located in the pia matter on the surface of the cortex and drain the cerebral cortex and subcortical white matter. The dural sinuses are therefore located on the surface of the cerebrum. The most prominent of these sinuses is the superior sagittal sinus which flows in the sagittal plane under the midline of the cerebral vault, posteriorly and inferiorly to the confluence of sinuses, where the superficial drainage joins with the sinus that primarily drains the deep venous system. From here, two transverse sinuses bifurcate and travel laterally and inferiorly in an S-shaped curve that form the sigmoid sinuses which go on to form the two jugular veins. In the neck, the jugular veins parallel the upward course of the carotid arteries and drain blood into the superior vena cava.
The deep or central veins consist of subependymal veins, internal cerebral veins, basal vein, and the great vein of Galen. These veins drain the brain’s interior, including the deep white and gray matter surrounding the lateral and third ventricles or the basal cistern and anastomose with the cortical veins, emptying into the superior sagittal sinus (SSS). Venous outflow from the SSS and deep veins is directed via a confluence of sinuses toward the sigmoid sinuses and jugular veins. The cerebellum is drained primarily by two sets of veins, the inferior cerebellar veins and the occipital sinuses. The brain stem is drained by the veins terminating in the inferior and transverse petrosal sinuses.
Figure 2. Venous circulation of the brain
Footnote: Illustrations depicting the predominant veins and sinuses involved in the craniocervical venous outflow. Venous narrowing is depicted at locations of interest in chronic cerebrospinal venous insufficiency (CCSVI).
Cerebrospinal fluid
Cerebrospinal fluid (CSF) is a clear, colorless liquid composed primarily of water that protects the brain and spinal cord from chemical and physical injuries. Cerebrospinal fluid also carries small amounts of oxygen, glucose, and other needed chemicals from the blood to neurons and neuroglia. CSF continuously circulates through cavities in the brain and spinal cord and around the brain and spinal cord in the subarachnoid space (the space between the arachnoid mater and pia mater). The total volume of CSF is 80 to 150 mL (3 to 5 oz) in an adult. CSF contains small amounts of glucose, proteins, lactic acid, urea, cations (Na+, K+, Ca2+, Mg2+), and anions (Cl− and HCO3 −); it also contains some white blood cells.
CSF functions
The CSF has three basic functions in helping to maintain homeostasis.
- Mechanical protection. CSF serves as a shock-absorbing medium that protects the delicate tissues of the brain and spinal cord from jolts that would otherwise cause them to hit the bony walls of the cranial cavity and vertebral canal. The fluid also buoys the brain so that it “floats” in the cranial cavity.
- Chemical protection. CSF provides an optimal chemical environment for accurate neuronal signaling. Even slight changes in the ionic composition of CSF within the brain can seriously disrupt production of action potentials and postsynaptic potentials.
- Circulation. CSF is a medium for minor exchange of nutrients and waste products between the blood and adjacent nervous tissue.
CSF formation
The majority of CSF production is from the choroid plexuses (membranelike), networks of blood capillaries in the walls of the ventricles. Ependymal cells joined by tight junctions cover the capillaries of the choroid plexuses. Selected substances (mostly water) from the blood plasma, which are filtered from the capillaries, are secreted by the ependymal cells to produce the cerebrospinal fluid. This secretory capacity is bidirectional and accounts for continuous production of CSF and transport of metabolites from the nervous tissue back to the blood. Because of the tight junctions between ependymal cells, materials entering CSF from choroid capillaries cannot leak between these cells; instead, they must pass through the ependymal cells. This blood–cerebrospinal fluid barrier permits certain substances to enter the CSF but excludes others, protecting the brain and spinal cord from potentially harmful blood-borne substances. In contrast to the blood–brain barrier (BBB), which is formed mainly by tight junctions of brain capillary endothelial cells, the blood–cerebrospinal fluid barrier is formed by tight junctions of ependymal cells.
Figure 3. CSF formation
Flow of CSF in brain
The CSF formed in the choroid plexuses of each lateral ventricle flows into the third ventricle through two narrow, oval openings, the interventricular foramina. More CSF is added by the choroid plexus in the roof of the third ventricle. The fluid then flows through the aqueduct of the midbrain (cerebral aqueduct), which passes through the midbrain, into the fourth ventricle. The choroid plexus of the fourth ventricle contributes more fluid. CSF enters the subarachnoid space through three openings in the roof of the fourth ventricle: a single median aperture and paired lateral apertures, one on each side. CSF then circulates in the central canal of the spinal cord and in the subarachnoid space around the surface of the brain and spinal cord.
CSF is gradually reabsorbed into the blood through arachnoid villi, fingerlike extensions of the arachnoid mater that project into the dural venous sinuses, especially the superior sagittal sinus. A cluster of arachnoid villi is called an arachnoid granulation. Normally, CSF is reabsorbed as rapidly as it is formed by the choroid plexuses, at a rate of about 20 mL/hr (480 mL/day). Because the rates of formation and reabsorption are the same, the pressure of CSF normally is constant. For the same reason, the volume of CSF remains constant. Figure 4 summarizes the production and flow of CSF.
Figure 4. CSF circulation
Figure 5. Cerebrospinal fluid formation, absorption and circulation around and within the brain
Figure 6. CSF absorption
- Heinrich Mattle & Marco Mumenthaler with Ethan Taub (2016-12-14). Fundamentals of Neurology. Thieme. p. 129. ISBN 978-3-13-136452-4.[↩]
- Kandel E.R., Schwartz, J.H., Jessell, T.M. 2000. Principles of Neural Science, 4th ed., McGraw-Hill, New York. p.1305[↩][↩]
- Blood gases. https://medlineplus.gov/ency/article/003855.htm[↩]
- Giardino ND, Friedman SD, Dager SR. Anxiety, respiration, and cerebral blood flow: implications for functional brain imaging. Compr Psychiatry 2007;48:103–112.[↩][↩]
- Cerebral Blood Flow (CBF). https://www.anaesthesiauk.com/article.aspx?articleid=100754[↩]
- Rhoton AL. The supratentorial arteries. Neurosurgery. 2002 Oct;51(4 Suppl):S53-120.[↩]
- Kiserud T, Acharya G. The fetal circulation. Prenat. Diagn. 2004 Dec 30;24(13):1049-59.[↩]
- Katz DA, Marks MP, Napel SA, Bracci PM, Roberts SL. Circle of Willis: evaluation with spiral CT angiography, MR angiography, and conventional angiography. Radiology. 1995 May;195(2):445-9.[↩]
- Konan LM, Mesfin FB. Neuroanatomy, Cerebral Blood Supply. [Updated 2019 Jan 17]. In: StatPearls [Internet]. Treasure Island (FL): StatPearls Publishing; 2019 Jan-. Available from: https://www.ncbi.nlm.nih.gov/books/NBK532297[↩][↩][↩]
- Vellore Y, Madan A, Hwang PY. Recurrent artery of Heubner aneurysm. Asian J Neurosurg. 2014;9(4):244. doi:10.4103/1793-5482.146658 https://www.ncbi.nlm.nih.gov/pmc/articles/PMC4323984[↩]
- Lama S, Dolati P, Sutherland GR. Controversy in the management of lenticulostriate artery dissecting aneurysm: a case report and review of the literature. World Neurosurg. 2014 Feb;81(2):441.e1-7.[↩]
- Tanriover N, Kucukyuruk B, Ulu MO, Isler C, Sam B, Abuzayed B, Uzan M, Ak H, Tuzgen S. Microsurgical anatomy of the cisternal anterior choroidal artery with special emphasis on the preoptic and postoptic subdivisions. J. Neurosurg. 2014 May;120(5):1217-28.[↩]
- Gunnal S, Farooqui M, Wabale R. Anatomical Variability in the Termination of the Basilar Artery in the Human Cadaveric Brain. Turk Neurosurg. 2015;25(4):586-94.[↩]
- Mehinovic A, Isakovic E, Delic J. Variations in diameters of vertebro-basilar tree in patients with or with no aneurysm. Med Arch. 2014;68(1):27-9.[↩]
- Kiliç T, Akakin A. Anatomy of cerebral veins and sinuses. Frontiers Neurol Neurosci. 2008; 23: pp. 4–15.[↩]