The nervous system
The nervous system has two major anatomical subdivisions:
- The central nervous system (CNS) consists of the brain and spinal cord, which are enclosed and protected by the cranium and vertebral column. The central nervous system is discussed further in the other posts: Human brain and Spinal cord.
- The peripheral nervous system (PNS) consists of all the rest; it is composed of nerves and ganglia. A nerve is a bundle of nerve fibers (axons) wrapped in fibrous connective tissue. Nerves emerge from the central nervous system (CNS) through foramina of the skull and vertebral column and carry signals to and from other organs of the body. A ganglion (plural, ganglia) is a knotlike swelling in a nerve where the cell bodies of peripheral neurons are concentrated.
Figure 1. Nervous system and its parts
Peripheral nervous system
The peripheral nervous system is functionally divided into sensory and motor divisions, and each of these is further divided into somatic and visceral subdivisions.
The sensory (afferent) division carries signals from various receptors (sense organs and simple sensory nerve endings) to the central nervous system (CNS). This pathway informs the central nervous system (CNS) of stimuli within and around the body.
- The somatic sensory division carries signals from receptors in the skin, muscles, bones, and joints.
- The visceral sensory division carries signals mainly from the viscera of the thoracic and abdominal cavities, such as the heart, lungs, stomach, and urinary bladder.
The motor (efferent) division carries signals from the CNS (the brain and the spinal cord) mainly to gland and muscle cells that carry out the body’s responses. Cells and organs that respond to these signals are called effectors.
- The somatic motor division carries signals to the skeletal muscles. This produces voluntary muscle contractions as well as involuntary somatic reflexes.
- The visceral motor division (autonomic nervous system) carries signals to glands, cardiac muscle, and smooth muscle. You usually have no voluntary control over these effectors, and the autonomic nervous system operates at an unconscious level. The responses of the autonomic nervous system and its effectors are visceral reflexes. The autonomic nervous system has two further divisions:
- The sympathetic division tends to arouse the body for action—for example, by accelerating the heartbeat and increasing respiratory airflow—but it inhibits digestion.
- The parasympathetic division tends to have a calming effect—slowing the heartbeat, for example—but it stimulates digestion.
Nervous system function
The communicative role of the nervous system is carried out by nerve cells, or neurons. These cells have three fundamental physiological properties that enable them to communicate with other cells:
- Excitability. All cells are excitable—that is, they respond to environmental changes (stimuli). Neurons exhibit this property to the highest degree.
- Conductivity. Neurons respond to stimuli by producing electrical signals that are quickly conducted to other cells at distant locations.
- Secretion. When the signal reaches the end of a nerve fiber, the neuron secretes a neurotransmitter that crosses the gap and stimulates the next cell.
Functional Classes of Neurons
There are three general classes of neurons corresponding to the three major aspects of nervous system function listed above (e.g. excitability, conductivity and secretion):
- Sensory (afferent) neurons are specialized to detect stimuli such as light, heat, pressure, and chemicals, and transmit information about them to the central nervous system (CNS). Such neurons begin in almost every organ of the body and end in the central nervous system (CNS); the word afferent refers to signal conduction toward the central nervous system (CNS). Some receptors, such as those for pain and smell, are themselves neurons. In other cases, such as taste and hearing, the receptor is a separate cell that communicates directly with a sensory neuron.
- Interneurons lie entirely within the central nervous system (CNS). They receive signals from many other neurons and carry out the integrative function of the nervous system—that is, they process, store, and retrieve information and “make decisions” that determine how the body responds to stimuli. About 90% of our neurons are interneurons. The word interneuron refers to the fact that they lie between, and interconnect, the incoming sensory pathways and the outgoing motor pathways of the central nervous system (CNS).
- Motor (efferent) neurons send signals predominantly to muscle and gland cells, the effectors. They are called motor neurons because most of them lead to muscle cells, and efferent neurons to signify signal conduction away from the central nervous system (CNS).
Figure 2. Functional classes of neurons
Structure of a Neuron
There are several varieties of neurons, but a good starting point for discussion is a motor neuron of the spinal cord. The control center of the neuron is the neurosoma, also called the soma or cell body. It has a centrally located nucleus with a large nucleolus. The cytoplasm contains mitochondria, lysosomes, a Golgi complex, numerous inclusions, and an extensive rough endoplasmic reticulum and cytoskeleton. The cytoskeleton consists of a dense mesh of microtubules and neurofibrils (bundles of actin filaments), which compartmentalize the rough endoplasmic reticulum into darkstaining regions called chromatophilic substance. This is unique to neurons and a helpful clue to identifying them in tissue sections with mixed cell types. Mature neurons have no centrioles and cannot undergo any further mitosis after adolescence. Consequently, neurons that die are usually irreplaceable; surviving neurons cannot multiply to replace those lost. However, neurons are unusually long-lived cells, capable of functioning for over a hundred years. But even in old age, there are unspecialized stem cells in some parts of the central nervous system (CNS) that can divide and regenerate nervous tissue to a limited extent.
The major inclusions in a neuron are glycogen granules, lipid droplets, melanin, and a golden brown pigment called lipofuscin, produced when lysosomes degrade worn-out organelles and other products. Lipofuscin accumulates with age and pushes the nucleus to one side of the cell. Lipofuscin granules are also called “wear-and-tear granules” because they are most abundant in old neurons. They seem harmless to neuron function.
Figure 3. General structure of a neuron
The somas of most neurons give rise to a few thick processes that branch into a vast number of dendrites—named for their striking resemblance to the bare branches of a tree in winter. Dendrites are the primary site for receiving signals from other neurons. Some neurons have only one dendrite and some have thousands. The more dendrites a neuron has, the more information it can receive and incorporate into its decision making. As tangled as the dendrites may seem, they provide exquisitely precise pathways for the reception and processing of neural information.
On one side of the neurosoma is a mound called the axon hillock, from which the axon (nerve fiber) originates. The axon is cylindrical and relatively unbranched for most of its length, although it may give rise to a few branches called axon collaterals near the soma, and most axons branch extensively at their distal end. An axon is specialized for rapid conduction of nerve signals to points remote from the soma. Its cytoplasm is called the axoplasm and its membrane the axolemma. A neuron never has more than one axon, and some neurons have none.
Somas range from 5 to 135 μm in diameter, and axons from 1 to 20 μm in diameter and from a few millimeters to more than a meter long. Such dimensions are more impressive when you scale them up to the size of familiar objects. If the soma of a spinal motor neuron were the size of a tennis ball, its dendrites would form a dense bushy mass that could fill a 30-seat classroom from floor to ceiling. Its axon would be up to a mile long but a little narrower than a garden hose. The neuron must assemble molecules and organelles in its “tennis ball” soma and deliver them through its “mile-long garden hose” to the end of the axon.
At the distal end, an axon usually has a terminal arborization—an extensive complex of fine branches. Each branch ends in a bulbous axon terminal (terminal button), which forms a junction (synapse) with the next cell. It contains synaptic vesicles full of neurotransmitter. In autonomic neurons, however, the axon has numerous beads called varicosities along its length. Each varicosity contains synaptic vesicles and secretes neurotransmitter.
Not all neurons fit the preceding description. Neurons are classified structurally according to the number of processes extending from the soma:
- Multipolar neurons are those, like the preceding, that have one axon and multiple dendrites. This is the most common type and includes most neurons of the brain and spinal cord.
- Bipolar neurons have one axon and one dendrite. Examples include olfactory cells of the nose, certain neurons of the retina, and sensory neurons of the ear.
- Unipolar neurons have only a single process leading away from the soma. They are represented by the neurons that carry signals to the spinal cord for such senses as touch and pain. They are also called pseudounipolar because they start out as bipolar neurons in the embryo, but their two processes fuse into one as the neuron matures. A short distance away from the soma, the process branches like a T into a peripheral fiber and a central fiber. The peripheral fiber begins with a sensory ending often far away from the soma—in the skin, for example. Its signals travel toward the soma, but bypass it and continue along the central fiber for a short remaining distance to the spinal cord. The dendrites are considered to be only the short receptive endings. The rest of the process, both peripheral and central, is the axon, defined by the presence of myelin and the ability to generate action potentials.
- Anaxonic neurons have multiple dendrites but no axon. They communicate locally through their dendrites and do not produce action potentials. Some anaxonic neurons are found in the brain, retina, and adrenal medulla. In the retina, they help in visual processes such as the perception of contrast.
Figure 4. Variation in Neuron Structure
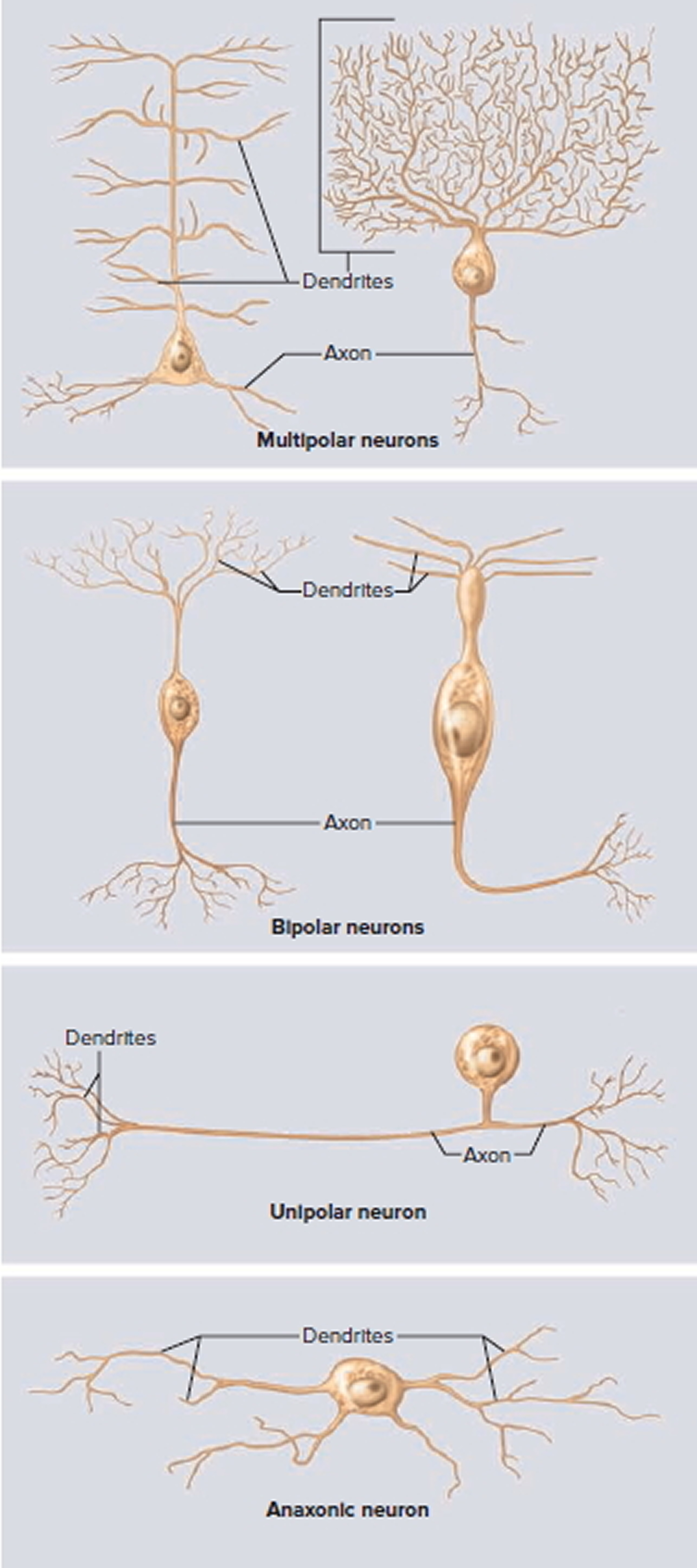
Note:
Top panel, left to right: Two multipolar neurons of the brain—a pyramidal cell and a Purkinje cell.
Second panel, left to right: Two bipolar neurons—a bipolar cell of the retina and an olfactory neuron.
Third panel: A unipolar neuron of the type involved in the senses of touch and pain.
Bottom panel: An anaxonic neuron (amacrine cell) of the retina.
Axonal Transport
All of the proteins needed by a neuron must be made in the soma, where the protein-synthesizing organelles such as the nucleus, ribosomes, and rough endoplasmic reticulum are located. Yet many of these proteins are needed in the axon, for example to repair and maintain the axolemma, to serve as ion channels in the membrane, or to act in the axon terminal as enzymes and signaling molecules. Other substances are transported from the axon terminals back to the soma for disposal or recycling. The two-way passage of proteins, organelles, and other materials along an axon is called axonal transport. Movement away from the soma down the axon is called anterograde transport and movement up the axon toward the soma is called retrograde transport.
Materials travel along axonal microtubules that act like monorail tracks to guide them to their destination. But what is the “engine” that drives them along the tracks ? Anterograde transport employs a motor protein called kinesin and retrograde transport uses one called dynein (the same protein responsible for the motility of cilia and flagella). These proteins carry materials “on their backs” while they reach out, like the myosin heads of muscle, to bind repeatedly to the microtubules and walk along them.
There are two types of axonal transport: fast and slow.
- Fast axonal transport occurs at a rate of 200 to 400 mm/day and may be either anterograde or retrograde:
+ Fast anterograde transport moves mitochondria; synaptic vesicles; other organelles; components of the axolemma; calcium ions; enzymes such as acetylcholinesterase; and small molecules such as glucose, amino acids, and nucleotides toward the distal end of the axon.
+ Fast retrograde transport returns used synaptic vesicles and other materials to the soma and informs the soma of conditions at the axon terminals. Some pathogens exploit this process to invade the nervous system. They enter the distal tips of an axon and travel to the soma by retrograde transport. Examples include tetanus toxin and the herpes simplex, rabies, and polio viruses. In such infections, the delay between infection and the onset of symptoms corresponds to the time needed for the pathogens to reach the somas. - Slow axonal transport is an anterograde process that works in a stop-and-go fashion. If you compare fast axonal transport to an express train traveling nonstop to its destination, slow axonal transport is like a local train that stops at every station. When moving, it goes just as fast as the express train, but the frequent stops result in an overall progress of only 0.2 to 0.5 mm/day. It moves enzymes and cytoskeletal components down the axon, renews worn-out axoplasmic components in mature neurons, and supplies new axoplasm for developing or regenerating neurons. Damaged nerves regenerate at a speed governed by slow axonal transport.
Supportive Cells (Neuroglia)
There are about a trillion (1,000,000,000,000 or 1012) neurons in the nervous system. Because they branch so extensively, they make up about 50% of the volume of the nervous tissue. Yet they are outnumbered at least 10 to 1 by cells called neuroglia or glial cells. Glial cells protect the neurons and help them function.
The word glia, which means “glue,” implies one of their roles—to bind neurons together and provide a supportive framework for the nervous tissue. In the fetus, they form a scaffold that guides young migrating neurons to their destinations. Wherever a mature neuron is not in synaptic contact with another cell, it is covered with glial cells. This prevents neurons from contacting each other except at points specialized for signal transmission, thus giving precision to their conduction pathways.
Figure 5. Neuroglia of the Central Nervous System
Types of Neuroglia
There are six kinds of neuroglia, each with a unique function. The first four types occur only in the central nervous system (brain and spinal cord):
- Oligodendrocytes somewhat resemble an octopus; they have a bulbous body with as many as 15 arms. Each arm reaches out to a nerve fiber and spirals around it like electrical tape wrapped repeatedly around a wire. This wrapping, called the myelin sheath, insulates the nerve fiber from the extracellular fluid. It speeds up signal conduction in the nerve fiber.
- Ependymal resemble a cuboidal epithelium lining the internal cavities of the brain and spinal cord. Unlike true epithelial cells, however, they have no basement membrane and they exhibit rootlike processes that penetrate into the underlying tissue. Ependymal cells produce cerebrospinal fluid (CSF), a liquid that bathes the central nervous system (brain and spinal cord) and fills its internal cavities. They have patches of cilia on their apical surfaces that help to circulate the cerebrospinal fluid.
- Microglia are small macrophages that develop from white blood cells called monocytes. They wander through the central nervous system (brain and spinal cord), putting out fingerlike extensions to constantly probe the tissue for cellular debris or other problems. They are thought to perform a complete checkup on the brain tissue several times a day, phagocytizing dead tissue, microorganisms, and other foreign matter. They become concentrated in areas damaged by infection, trauma, or stroke. Pathologists look for clusters of microglia in brain tissue as a clue to sites of injury. Microglia also aid in synaptic remodeling, changing the connections between neurons.
- Astrocytes are the most abundant glial cells in the central nervous system (brain and spinal cord) and constitute over 90% of the tissue in some areas of the brain. They cover the entire brain surface and most nonsynaptic regions of the neurons in the gray matter. They are named for their many-branched, somewhat starlike shape. They have the most diverse functions of any glia:
- + They form a supportive framework for the nervous tissue.
- + They have extensions called perivascular feet, which contact the blood capillaries and stimulate them to form a tight, protective seal called the blood–brain barrier.
- + They monitor neuron activity, stimulate dilation and constriction of blood vessels, and thus regulate blood flow in the brain tissue to meet changing needs for oxygen and nutrients.
- + They convert blood glucose to lactate and supply this to the neurons for nourishment.
- + They secrete nerve growth factors that regulate nerve development.
- + They communicate electrically with neurons and influence synaptic signaling between them.
- + They regulate the composition of the tissue fluid. When neurons transmit signals, they release neurotransmitters and potassium ions. Astrocytes absorb these and prevent them from reaching excessive levels in the tissue fluid.
- + When neurons are damaged, astrocytes form hardened scar tissue and fill space formerly occupied by the neurons. This process is called astrocytosis or sclerosis.
The other two types of glial cells occur only in the peripheral nervous system:
- Schwann cells or neurilemmocytes, envelop nerve fibers of the peripheral nervous system. In most cases, a Schwann cell winds repeatedly around a nerve fiber and produces a myelin sheath similar to the one produced by oligodendrocytes in the central nervous system (brain and spinal cord). There are some important differences in myelin production between the central nervous system (brain and spinal cord) and peripheral nervous system, which we consider shortly. Schwann cells also assist in the regeneration of damaged fibers, as described later.
- Satellite cells surround the somas in ganglia of the peripheral nervous system. They provide insulation around the soma and regulate the chemical environment of the neurons.
Myelin
The myelin sheath is a spiral layer of insulation around a nerve fiber, formed by oligodendrocytes in the central nervous system (brain and spinal cord) and Schwann cells in the peripheral nervous system. Since it consists of the plasma membranes of glial cells, its composition is like that of plasma membranes in general. It is about 20% protein and 80% lipid, the latter including phospholipids, glycolipids, and cholesterol.
Production of the myelin sheath is called myelination. It begins in the fourteenth week of fetal development, yet hardly any myelin exists in the brain at the time of birth. Myelination proceeds rapidly in infancy and isn’t completed until late adolescence. Since myelin has such a high lipid content, dietary fat is important to early nervous system development. It is best not to give children under 2 years old the sort of low-fat diets (skimmed milk, etc.) that may be beneficial to an adult.
Figure 6. Myelination
Note: (a) A Schwann cell of the peripheral nervous system, wrapping repeatedly around an axon to form the multilayered myelin sheath.
The myelin spirals outward away from the axon as it is laid down.
(b) An oligodendrocyte of the central nervous system (brain and spinal cord) wrapping around the axons of multiple neurons. Here, the myelin spirals inward toward the axon as it is laid down.
(c) A myelinated axon (top) and unmyelinated axon (bottom).
In the peripheral nervous system, a Schwann cell spirals repeatedly around a single nerve fiber, laying down up to 100 compact layers of its own membrane with almost no cytoplasm between the membranes. These layers constitute the myelin sheath. The Schwann cell spirals outward as it wraps the nerve fiber, finally ending with a thick outermost coil called the neurilemma. Here, the bulging body of the Schwann cell contains its nucleus and most of its cytoplasm. External to the neurilemma is a basal lamina and then a thin sleeve of fibrous connective tissue called the endoneurium. To visualize this myelination process, imagine that you wrap an almost-empty tube of toothpaste tightly around a pencil. The pencil represents the axon, and the spiral layers of toothpaste tube represent the myelin. The toothpaste, like the cytoplasm of the cell, would be forced to one end of the tube and form a bulge on the external surface of the wrapping, like the body of the Schwann cell.
In the central nervous system (brain and spinal cord), each oligodendrocyte reaches out to myelinate several nerve fibers in its immediate vicinity. Since it is anchored to multiple nerve fibers, it cannot migrate around any one of them like a Schwann cell does. It must push newer layers of myelin under the older ones, so myelination spirals inward toward the nerve fiber. Nerve fibers of the central nervous system (brain and spinal cord) have no neurilemma or endoneurium. The contrasting modes of myelination are called centrifugal myelination (“away from the center”) in the peripheral nervous system and centripetal myelination (“toward the center”) in the central nervous system (brain and spinal cord).
In both the peripheral nervous system and central nervous system (brain and spinal cord), a nerve fiber is much longer than the reach of a single glial cell, so it requires many Schwann cells or oligodendrocytes to cover one nerve fiber. Consequently, the myelin sheath is segmented. Each gap between segments is called a node of Ranvier or myelin sheath gap; the myelin-covered segments from each node to the next are called internodes. The internodes are about 0.2 to 1.0 mm long. The short section of nerve fiber between the axon hillock and the first glial cell is called the initial segment. Since the axon hillock and initial segment play an important role in initiating a nerve signal, they are collectively called the trigger zone.
Unmyelinated Nerve Fibers
Many nerve fibers in the peripheral nervous system and central nervous system (brain and spinal cord) are unmyelinated. In the peripheral nervous system, however, even the unmyelinated fibers are enveloped in Schwann cells. In this case, one Schwann cell harbors from 1 to 12 small nerve fibers in grooves in its surface. The Schwann cell’s plasma membrane does not spiral repeatedly around the fiber as it does in a myelin sheath, but folds once around each fiber and may somewhat overlap itself along the edges. This wrapping is the neurilemma. Most nerve fibers travel through individual channels in the Schwann cell, but small fibers are sometimes bundled together within a single channel. A basal lamina surrounds the entire Schwann cell along with its nerve fibers.
Figure 7. Unmyelinated nerve fibers
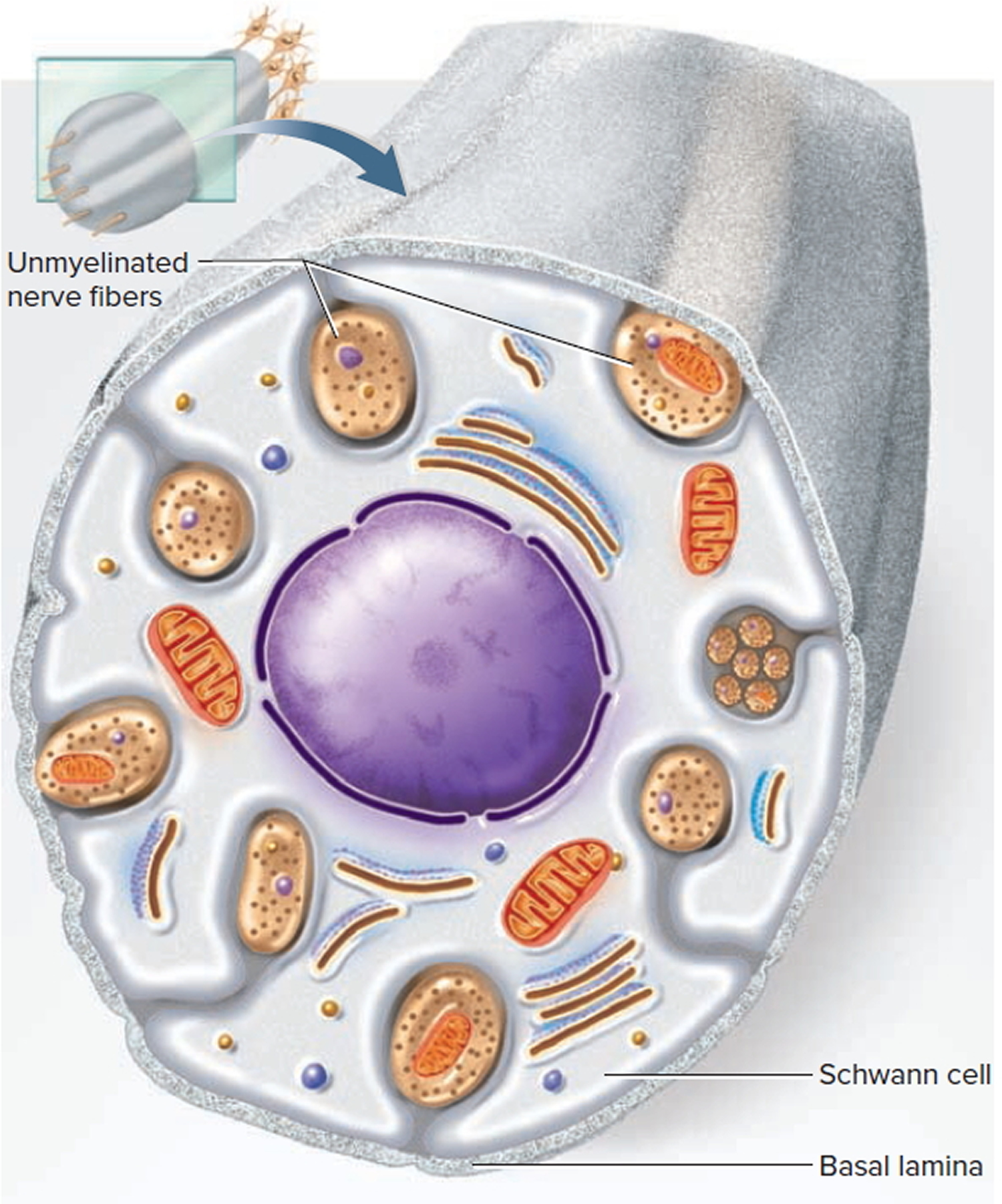
Conduction Speed of Nerve Fibers
The speed at which a nerve signal travels along a nerve fiber depends on two factors: the diameter of the fiber and the presence or absence of myelin. Signal conduction occurs along the surface of a fiber, not deep within its axoplasm. Large fibers have more surface area and conduct signals more rapidly than small fibers.
Myelin further speeds signal conduction. Nerve signals travel about 0.5 to 2.0 m/s in small unmyelinated fibers (2–4 μm in diameter); 3 to 15 m/s in myelinated fibers of the same size; and as fast as 120 m/s in large myelinated fibers (up to 20 μm in diameter). You might wonder why all of your nerve fibers are not large, myelinated, and fast; but if this were so, your nervous system would be impossibly bulky or limited to far fewer fibers. Large nerve fibers require large somas and a large expenditure of energy to maintain them. The evolution of myelin allowed for the subsequent evolution of more complex and responsive nervous systems with smaller, more energy-efficient neurons. Slow unmyelinated fibers are quite sufficient for processes in which quick responses are not particularly important, such as secreting stomach acid or dilating the pupil. Fast myelinated fibers are employed where speed is more important, as in motor commands to the skeletal muscles and sensory signals for vision and balance.
Nerve Regeneration
Nerve fibers of the peripheral nervous system are vulnerable to cuts, crushing injuries, and other trauma. A damaged peripheral nerve fiber may regenerate, however, if its soma is intact and at least some neurilemma remains. Figure 8 shows the process of regeneration, taking as its example a somatic motor neuron:
Figure 8. Regeneration of a Damaged Nerve Fiber
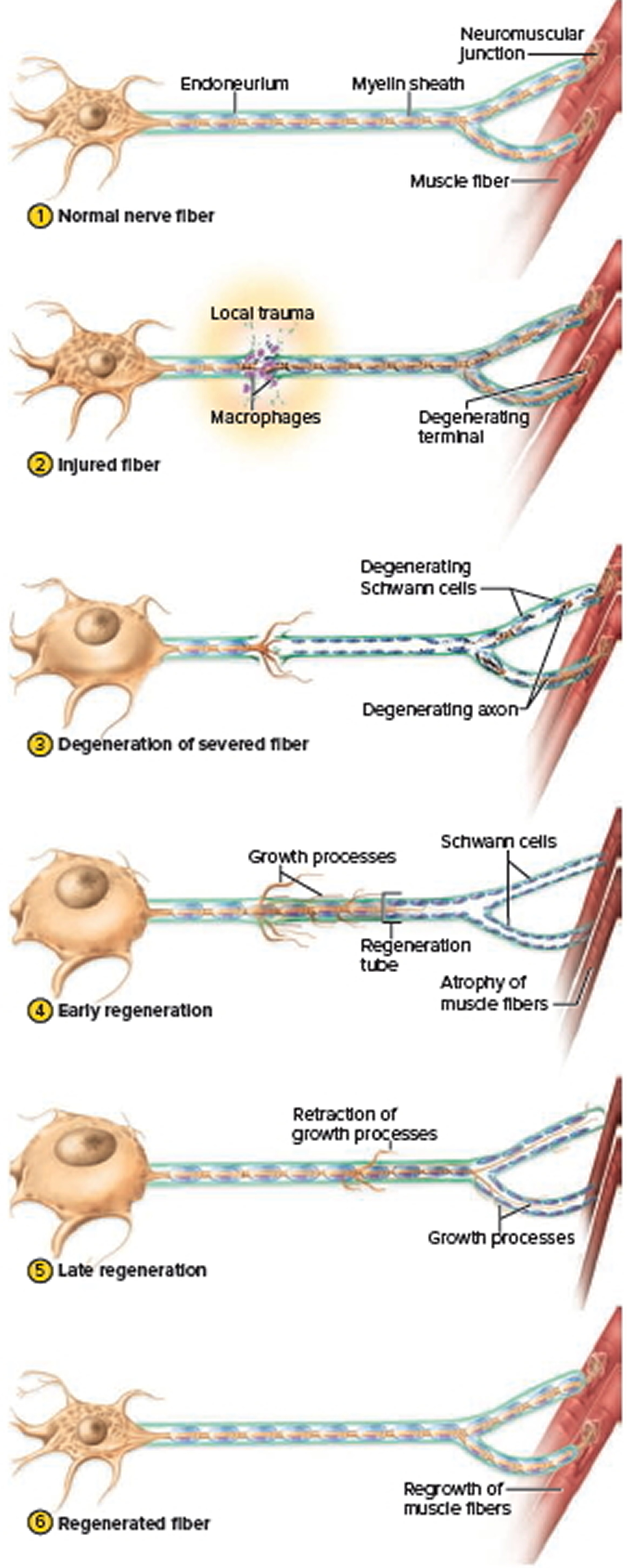
- In the normal nerve fiber, note the size of the soma and the size of the muscle fibers for comparison to later stages.
- When a nerve fiber is cut, the fiber distal to the injury cannot survive because it is incapable of protein synthesis. Protein-synthesizing organelles are mostly in the soma. As the distal fiber degenerates, so do its Schwann cells, which depend on it for their maintenance. Macrophages clean up tissue debris at the point of injury and beyond.
- The soma exhibits a number of abnormalities of its own, probably because it is cut off from the supply of nerve growth factors from the neuron’s target cells. The soma swells, the endoplasmic reticulum breaks up (so the chromatophilic substance disperses), and the nucleus moves off center. Not all damaged neurons survive; some die at this stage. But often, the axon stump sprouts multiple growth processes as the severed distal end shows continued degeneration of its axon and Schwann cells. Muscle fibers deprived of their nerve supply exhibit a shrinkage called denervation atrophy.
- Near the injury, Schwann cells, the basal lamina, and the neurilemma form a regeneration tube. The Schwann cells produce cell-adhesion molecules and nerve growth factors that enable a neuron to regrow to its original destination. When one growth process finds its way into the tube, it grows rapidly (3–5 mm/day), and the other growth processes are retracted.
- The regeneration tube guides the growing sprout back to the original target cells, reestablishing synaptic contact.
- When contact is established, the soma shrinks and returns to its original appearance, and the reinnervated muscle fibers regrow. Regeneration is not perfect. Some nerve fibers connect to the wrong muscle fibers or never find a muscle fiber at all, and some damaged motor neurons simply die. Nerve injury is therefore often followed by some degree of functional deficit. Even when regeneration is achieved, the slow rate of axon regrowth means that some nerve function may take as long as 2 years to recover. Schwann cells and endoneurium are required for nerve fiber regeneration. Both of these are lacking from the central nervous system (brain and spinal cord), so damaged central nervous system (brain and spinal cord) nerve fibers cannot regenerate at all. However, since the central nervous system (brain and spinal cord) is encased in bone, it suffers less trauma than the peripheral nerves.
Nervous system diseases
There are more than 600 neurologic diseases 1. Major types include:
- Diseases caused by faulty genes, such as Huntington’s disease and muscular dystrophy
- Problems with the way the nervous system develops, such as spina bifida
- Degenerative diseases, where nerve cells are damaged or die, such as Parkinson’s disease and Alzheimer’s disease
- Diseases of the blood vessels that supply the brain, such as stroke
- Injuries to the spinal cord and brain
- Seizure disorders, such as epilepsy
- Cancer, such as brain tumors
- Infections, such as meningitis.
Alzheimer’s disease
Alzheimer’s disease is the most common form of dementia among older people 2. Dementia is a brain disorder that seriously affects a person’s ability to carry out daily activities. Alzheimer’s disease is the fourth leading cause of death in adults 3. The incidence of the disease rises steeply with age. Alzheimer’s disease is twice as common in women than in men.
Alzheimer’s disease tends to run in families; currently, mutations in four genes, situated on chromosomes 1, 14, 19, and 21, are believed to play a role in the disease 3.
Alzheimer’s disease begins slowly. It first involves the parts of the brain that control thought, memory and language. People with Alzheimer’s disease may have trouble remembering things that happened recently or names of people they know. A related problem, mild cognitive impairment, causes more memory problems than normal for people of the same age. Many, but not all, people with mild cognitive impairment will develop Alzheimer’s disease.
In Alzheimer’s disease, over time, symptoms get worse. People may not recognize family members. They may have trouble speaking, reading or writing. They may forget how to brush their teeth or comb their hair. Later on, they may become anxious or aggressive, or wander away from home. Eventually, they need total care. This can cause great stress for family members who must care for them.
Alzheimer’s disease usually begins after age 60. The risk goes up as you get older. Your risk is also higher if a family member has had the disease.
No treatment can stop the disease. However, some drugs may help keep symptoms from getting worse for a limited time.
Epilepsy
Epilepsy is a brain disorder that causes people to have recurring seizures 4. The seizures happen when clusters of nerve cells, or neurons, in the brain send out the wrong signals. People may have strange sensations and emotions or behave strangely. They may have violent muscle spasms or lose consciousness.
Epilepsy affects approximately 1% of the population making it one of the most common neurological diseases 5. Epilepsy can strike at any time of life—from infancy to old age. There are many forms of epilepsy—most are rare. While epilepsy varies widely in type and severity, all forms of this disorder are characterized by recurring seizures resulting from abnormal cell firing in the brain. In approximately 30% of cases, epilepsy is caused by such events as head trauma, tumor, stroke, or infection. In those cases for which there is no known cause, recent evidence suggests there may be genetic predisposition to developing the disease.
Epilepsy has many possible causes, including illness, brain injury, and abnormal brain development. In many cases, the cause is unknown. But to date, at least twelve forms of epilepsy have been demonstrated to possess some genetic basis 5.
Doctors use brain scans and other tests to diagnose epilepsy. It is important to start treatment right away. There is no cure for epilepsy, but medicines can control seizures for most people. When medicines are not working well, surgery or implanted devices such as vagus nerve stimulators may help. Special diets can help some children with epilepsy.
Huntington’s disease
Huntington disease is an inherited, degenerative neurological disease that leads to dementia 6. Huntington’s disease causes certain nerve cells in the brain to waste away. People are born with the defective gene, but symptoms usually don’t appear until middle age. Early symptoms of Huntington’s disease may include uncontrolled movements, clumsiness, and balance problems. Later, Huntington’s disease can take away the ability to walk, talk, and swallow. Some people stop recognizing family members. Others are aware of their environment and are able to express emotions.
About 30,000 Americans have Huntington disease and about 150,000 more are at risk of inheriting the disease from a parent.
The Huntington disease gene, whose mutation results in Huntington disease, was mapped to chromosome 4 in 1983. The mutation is a characteristic expansion of a nucleotide triplet repeat in the DNA that codes for the protein huntingtin. As the number of repeated triplets – CAG (cytosine, adenine, guanine) – increases, the age of onset in the patient decreases. Furthermore, because the unstable trinucleotide repeat can lengthen when passed from parent to child, the age of onset can decrease from one generation to the next. Since people who have those repeats always suffer from Huntington disease, it suggests that the mutation causes a gain-of-function, in which the mRNA or protein takes on a new property or is expressed inappropriately.
If one of your parents has Huntington’s disease, you have a 50 percent chance of getting it. A blood test can tell you if have the Huntington’s disease gene and will develop the disease. Genetic counseling can help you weigh the risks and benefits of taking the test.
There is no cure. Medicines can help manage some of the symptoms, but cannot slow down or stop the disease.
Meningitis
Meningitis is inflammation of the thin tissue that surrounds the brain and spinal cord, called the meninges 7. There are several types of meningitis. The most common is viral meningitis. You get it when a virus enters the body through the nose or mouth and travels to the brain. Viral meningitis is almost never life-threatening and viruses rarely cause septicaemia 8. Bacterial meningitis is rare, but can be deadly. It usually starts with bacteria that cause a cold-like infection. It can cause stroke, hearing loss, and brain damage. It can also harm other organs. Pneumococcal infections and meningococcal infections are the most common causes of bacterial meningitis. Fungal meningitis is very rare, but is serious. Fungal meningitis usually only affects people with weakened immune systems.
Anyone can get meningitis, but it is more common in people with weak immune systems. Meningitis can get serious very quickly – it can kill in hours. You should get medical care right away if you have 7:
- A sudden high fever
- A severe headache
- A stiff neck
- Nausea or vomiting
Being able to recognise the symptoms of meningitis and septicaemia is vital because early recognition and treatment provide the best chance of a good recovery. Septicaemia is blood poisoning caused by the same germs that can cause meningitis.
Early treatment can help prevent serious problems, including death. Tests to diagnose meningitis include blood tests, imaging tests, and a spinal tap to test cerebrospinal fluid. Antibiotics can treat bacterial meningitis. Antiviral medicines may help some types of viral meningitis. Other medicines can help treat symptoms.
There are vaccines to prevent some of the bacterial infections that cause meningitis.
However, there are still some causes of meningitis and septicaemia which are not vaccine preventable and some vaccines are not routinely provided in some parts of the world.
Muscular dystrophy
Muscular dystrophy is a group of more than 30 inherited diseases. They all cause muscle weakness and muscle loss 9. In muscular dystrophy, abnormal genes (mutations) interfere with the production of proteins needed to form healthy muscle. Some forms of muscular dystrophy appear in infancy or childhood. Others may not appear until middle age or later. The different types can vary in whom they affect, which muscles they affect, and what the symptoms are. All forms of muscular dystrophy grow worse as the person’s muscles get weaker. Most people with muscular dystrophy eventually lose the ability to walk 9. Some may have trouble breathing or swallowing 10.
Duchenne muscular dystrophy
About half of people with muscular dystrophy have this variety 10. Although girls can be carriers and mildly affected, the disease typically affects boys.
About one-third of boys with Duchenne muscular dystrophy don’t have a family history of the disease, possibly because the gene involved may be subject to sudden abnormal change (spontaneous mutation).
Signs and symptoms typically appear between the ages of 2 and 3, and may include:
- Frequent falls
- Difficulty getting up from a lying or sitting position
- Trouble running and jumping
- Waddling gait
- Walking on the toes
- Large calf muscles
- Muscle pain and stiffness
- Learning disabilities
Becker muscular dystrophy
Signs and symptoms are similar to those of Duchenne muscular dystrophy, but typically are milder and progress more slowly. Symptoms generally begin in the teens but may not occur until the mid-20s or even later.
Other types of muscular dystrophy
Some types of muscular dystrophy are defined by a specific feature or by where in the body symptoms first begin. Examples include:
- Myotonic. Also known as Steinert’s disease, this form is characterized by an inability to relax muscles at will following contractions. Myotonic muscular dystrophy is the most common form of adult-onset muscular dystrophy. Facial and neck muscles are usually the first to be affected.
- Facioscapulohumeral (FSHD). Muscle weakness typically begins in the face and shoulders. The shoulder blades might stick out like wings when a person with FSHD raises his or her arms. Onset usually occurs in the teenage years but may begin in childhood or as late as age 40.
- Congenital. This type affects boys and girls and is apparent at birth or before age 2. Some forms progress slowly and cause only mild disability, while others progress rapidly and cause severe impairment.
- Limb-girdle. Hip and shoulder muscles are usually the first affected. People with this type of muscular dystrophy may have difficulty lifting the front part of the foot and so may trip frequently. Onset usually begins in childhood or the teenage years.
There is no cure for muscular dystrophy. Treatments can help with the symptoms and prevent complications. They include physical and speech therapy, orthopedic devices, surgery, and medications. Some people with muscular dystrophy have mild cases that worsen slowly. Others cases are disabling and severe.
Parkinson’s disease
Parkinson’s disease is a type of movement disorder. It happens when nerve cells in the brain don’t produce enough of a brain chemical called dopamine 11. Sometimes it is genetic, but most cases do not seem to run in families. Exposure to chemicals in the environment might play a role.
Symptoms begin gradually, often on one side of the body. Later they affect both sides. They include 11:
- Trembling of hands, arms, legs, jaw and face
- Stiffness of the arms, legs and trunk
- Slowness of movement
- Poor balance and coordination
As symptoms get worse, people with the disease may have trouble walking, talking, or doing simple tasks. They may also have problems such as depression, sleep problems, or trouble chewing, swallowing, or speaking.
There is no lab test for Parkinson’s disease, so it can be difficult to diagnose. Doctors use a medical history and a neurological examination to diagnose it.
Parkinson’s disease usually begins around age 60, but it can start earlier. It is more common in men than in women. There is no cure for Parkinson’s disease. A variety of medicines sometimes help symptoms dramatically. Surgery and deep brain stimulation can help severe cases. With deep brain stimulation, electrodes are surgically implanted in the brain. They send electrical pulses to stimulate the parts of the brain that control movement.
Spina bifida
Spina bifida is a neural tube defect – a type of birth defect of the brain, spine, or spinal cord. It happens if the spinal column of the fetus doesn’t close completely during the first month of pregnancy 12. Normally, the neural tube forms early in the pregnancy and closes by the 28th day after conception. In babies with spina bifida, a portion of the neural tube fails to develop or close properly, causing defects in the spinal cord and in the bones of the spine. This can damage the nerves and spinal cord. Screening tests during pregnancy can check for spina bifida. Sometimes it is discovered only after the baby is born.
The symptoms of spina bifida vary from person to person. Most people with spina bifida are of normal intelligence. Some people need assistive devices such as braces, crutches, or wheelchairs. They may have learning difficulties, urinary and bowel problems, or hydrocephalus, a buildup of fluid in the brain.
The exact cause of spina bifida is unknown. It seems to run in families. Taking folic acid can reduce the risk of having a baby with spina bifida. It’s in most multivitamins. Women who could become pregnant should take it daily.
Spina bifida occurs in various forms of severity. When treatment for spina bifida is necessary, it’s done surgically, although such treatment doesn’t always completely resolve the problem.
Stroke
A stroke is a medical emergency. Strokes happen when blood flow to your brain stops 13. Within minutes, brain cells begin to die. There are two kinds of stroke. The more common kind, called ischemic stroke, is caused by a blood clot that blocks or plugs a blood vessel in the brain. The other kind, called hemorrhagic stroke, is caused by a blood vessel that breaks and bleeds into the brain. “Mini-strokes” or transient ischemic attacks (TIAs), occur when the blood supply to the brain is briefly interrupted.
Symptoms of stroke are 13, 14:
- Sudden numbness or weakness of the face, arm or leg (especially on one side of the body). You may develop sudden numbness, weakness or paralysis in your face, arm or leg, especially on one side of your body. Try to raise both your arms over your head at the same time. If one arm begins to fall, you may be having a stroke. Similarly, one side of your mouth may droop when you try to smile.
- Sudden confusion, trouble speaking or understanding speech. You may experience confusion. You may slur your words or have difficulty understanding speech.
- Sudden trouble seeing in one or both eyes. You may suddenly have blurred or blackened vision in one or both eyes, or you may see double.
- Sudden trouble walking, dizziness, loss of balance or coordination. You may stumble or experience sudden dizziness, loss of balance or loss of coordination.
- Sudden severe headache with no known cause. A sudden, severe headache, which may be accompanied by vomiting, dizziness or altered consciousness, may indicate you’re having a stroke.
Seek immediate medical attention if you notice any signs or symptoms of a stroke, even if they seem to fluctuate or disappear. Call your local emergency number right away. Don’t wait to see if symptoms go away. Every minute counts. The longer a stroke goes untreated, the greater the potential for brain damage and disability.
If you’re with someone you suspect is having a stroke, watch the person carefully while waiting for emergency assistance.
If you have any of these symptoms, you must get to a hospital quickly to begin treatment. Acute stroke therapies try to stop a stroke while it is happening by quickly dissolving the blood clot or by stopping the bleeding. Post-stroke rehabilitation helps individuals overcome disabilities that result from stroke damage. Drug therapy with blood thinners is the most common treatment for stroke.
- Neurologic Diseases. Medline Plus. https://medlineplus.gov/neurologicdiseases.html[↩]
- Alzheimer’s Disease. Medline Plus. https://medlineplus.gov/alzheimersdisease.html[↩]
- National Center for Biotechnology Information (US). Genes and Disease [Internet]. Bethesda (MD): National Center for Biotechnology Information (US); 1998-. Alzheimer disease. Available from: https://www.ncbi.nlm.nih.gov/books/NBK22170/[↩][↩]
- Epilepsy. Medline Plus. https://medlineplus.gov/epilepsy.html[↩]
- National Center for Biotechnology Information (US). Genes and Disease [Internet]. Bethesda (MD): National Center for Biotechnology Information (US); 1998-. Epilepsy. Available from: https://www.ncbi.nlm.nih.gov/books/NBK22237/[↩][↩]
- National Center for Biotechnology Information (US). Genes and Disease [Internet]. Bethesda (MD): National Center for Biotechnology Information (US); 1998-. Huntington disease. Available from: https://www.ncbi.nlm.nih.gov/books/NBK22226/[↩]
- Meningitis. Medline Plus. https://medlineplus.gov/meningitis.html[↩][↩]
- What are meningitis and septicaemia ? Meningitis Research Foundation. https://www.meningitis.org/meningitis/what-is-meningitis[↩]
- Muscular Dystrophy. Medline Plus. https://medlineplus.gov/musculardystrophy.html[↩][↩]
- Muscular dystrophy. Mayo Clinic. http://www.mayoclinic.org/diseases-conditions/muscular-dystrophy/basics/definition/CON-20021240?p=1[↩][↩]
- Parkinson’s Disease. Medline Plus. https://medlineplus.gov/parkinsonsdisease.html[↩][↩]
- Spina Bifida. Medline Plus. https://medlineplus.gov/spinabifida.html[↩]
- Stroke. Medline Plus. https://medlineplus.gov/stroke.html[↩][↩]
- Stroke. Mayo Clinic. http://www.mayoclinic.org/diseases-conditions/stroke/symptoms-causes/syc-20350113[↩]