Xenoestrogens
Xenoestrogens also called endocrine disrupting chemicals or endocrine disruptors, are those chemicals that may act as inappropriate estrogens, and/or could interfere with the actions of endogenous estrogens, consequently acting as carcinogen (substance that causes cancer) 1. Xenoestrogens have wide structural diversity, but all have in common lipophillic phenolic rings and other hydrophobic components, a characteristic they share with steroid hormones and related nuclear receptor-activating compounds (see Figure 1). It has been suggested that the “promiscuity” of estrogen receptors (ERs) in accepting many diverse compounds may be due to their status as the most evolutionarily primitive versions of ligand-activatable regulatory proteins 2; as such they probably initially evolved to respond to a diverse set of molecules in the cell’s environment. Therefore, many compounds that are byproducts of our modern industrialized life-style (pesticides, herbicides, plastics manufacturing byproducts, fungicides, cosmetics additives, and pharmaceuticals) can serve as xenoestrogens in an inappropriate way.
Xenoestrogens are natural or industrial compounds found in the diet and environment that are capable of mimicking part of the effects of endogenous estrogens or interfering with estrogen signaling pathways by binding to estrogen receptors in the human body 3. This has consequence for estrogen-dependent health outcomes which includes reproductive health, puberty and pregnancy 4. A less commonly known xenoestrogen, Triclosan (5-chloro-2-(2,4-dichlorophenoxy) phenol) is a broad-spectrum antibacterial, mostly used in dentifrices (pastes or powders for cleaning the teeth), cosmetics, soap and other end user products 5. The detection of triclosan in human breast milk, urine and serum due to its extensive use is alarming due to its association with numerous health outcomes, including cancer development 5.
Many xenoestrogens are synthetic estrogens stemming from human activity, which, due to their use, can enter in contact with living organisms or be released into the environment. For instance, workers in the production of contraceptive pills were exposed to the potent estrogen ethinyl-estradiol (EE2) which is capable of being absorbed by the skin 6. A correlation was also established between the massive exposure to pesticide DDT (dichlorodiphenyltrichloroethane) by farm laborers and the risks of oligospermia 7. There is also the notorious example of diethylstilbestrol (DES), considered at its discovery as a miracle pill to fight against miscarriages and widely prescribed to pregnant women in the 70’s in France. Exposure to this chemical in utero induced serious deformities and disorders in the reproductive system of male and female children 8. More recently, an epidemiological study performed in the French West Indies analyzed the relationship between exposure to chlordecone and the risk of prostate cancer 9. Chlordecone is an insecticide which exhibits estrogenic-like activity, that was used extensively (from 1973 to 1993) to control the banana root borer, thus contaminating the foodstuffs and population for several years. Interestingly, this study showed a significant increase in the risk of prostate cancer as the plasma chlordecone concentration increased 9. Of course, these molecules are only a few of the numerous molecules whom estrogenic activities have been demonstrated or are suspected.
Furthermore, xenoestrogens are not only synthetic compounds, but there are also numerous natural molecules in food that exhibit estrogen-mimetic activities (see Figure 1 below). These natural molecules are mainly phytoestrogens isoflavones, and the most consumed are genistein and daidzein; in particular, these two xenoestrogens are contained in the subproducts of soy and some legumes, fruits, and nuts. Other groups of phytoestrogens such as flavones, coumestans, and lignans were also found 10. Additionally, certain mushrooms, mosses, or fungi can contain estrogen-like compounds called mycoestrogens, such as zearalenone 10.
Xenoestrogens, such as phthalates, can be extremely persistent in the environment. Some endocrine disrupting chemicals, for example, polychlorinated biphenyls (PCBs), are able to bioaccumulate in the food chain or in several biological matrices (as fats) and often exhibit effects at weak concentrations or in combination 11. Among the numerous sources of exposure, the ingestion of water or contaminated food, cosmetics, pharmaceuticals, industrial exposure, and contact via professional activities (e.g., pesticides) are the most common. It is important to underline that the exposure to these compounds can have particularly critical effects at the fetal and postnatal stages 12. The development of the nervous system and the reproductive organs can be severely disrupted at these stages, as numerous tissues are particularly sensitive to hormonal
regulation.
Figure 1. Xenoestrogens
[Source 13 ]Xenoestrogen sources
The origin and the exposure sources of xenoestrogens are multiple. They can come from food, products of combustion, and agricultural and industrial chemicals. Xenoestrogens are mostly found in plastic bottles, personal care products and pesticides 3.
Industrial pollutants which include heavy metals and dioxins showed estrogen-like activity. Fried meat consumers are also known to have a higher incidence of pancreatic cancer progression which might be associated with exposure to benzo(a)pyrene and different food contaminants that exhibit xenoestrogenic characteristics. Increase in brain cancer incidence was observed due to polycyclic aromatic hydrocarbons which are xenoestrogen-like agents. The susceptibility to xenoestrogens may differ which may be linked to steroid and xenobiotic receptor numbers, which are greater in young adults (15-38 years old) and reduced with the age 14.
Currently cancer risk estimation in humans does not give proper description for environmental and transplacental exposure to xenoestrogens. The analysis of xenoestrogens role in the development of cancer must be done with a new approach in order point towards the complexity of carcinogenesis 14.
In 2003, it was reported that in breast cancer development, over 160 xenoestrogens were found responsible 15. Women were reported at higher risk of miscarriage, breast cancer, diabetes and more due to these xenoestrogens which are commonly found in makeup, lotions, pharmaceuticals (birth control), deodorants, fingernail polish and sunscreen. They were also reported in phthalates (plasticizers) and building supplies which include, electrical oils, wood preservatives and adhesives 16.
Figure 2. Sources of exposure to xenoestrogens
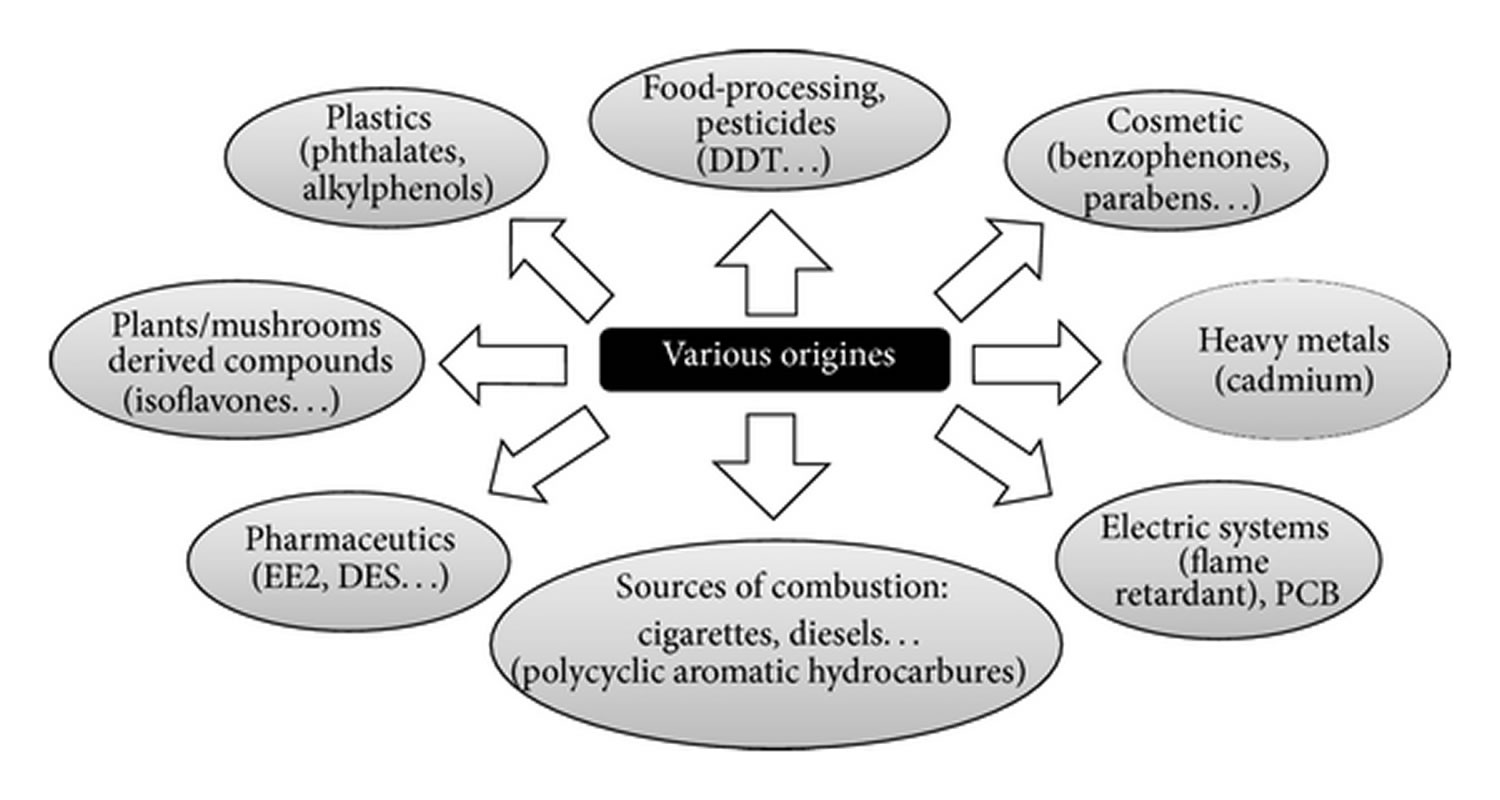
Xenoestrogens list
Here are some of the chemicals that are xenoestrogens:
Skincare:
- 4-Methylbenzylidene camphor (4-MBC) (sunscreen lotions)
- Parabens (methylparaben, ethylparaben, propylparaben and butylparaben commonly used as a preservative)
- Benzophenone (sunscreen lotions)
Industrial products and plastics:
- Bisphenol A (monomer for polycarbonate plastic and epoxy resin; antioxidant in plasticizers)
- Phthalates (plasticizers)
- Dioxin, a group of highly toxic chemicals are released during combustion processes, pesticide manufacturing and chlorine bleaching of wood pulp.
- DEHP (plasticizer for PVC)
- Polybrominated biphenyl ethers (PBDEs) (flame retardants used in plastics, foams, building materials, electronics, furnishings, motor vehicles).
- Polychlorinated biphenyls (PCBs)
- PBB (Polybrominated biphenyls)
Food:
- Erythrosine / FD&C Red No. 3
- Phenosulfothiazine (a red dye)
- Butylated hydroxyanisole / BHA (food preservative)
Building supplies:
- Pentachlorophenol (general biocide and wood preservative)
- Polychlorinated biphenyls / PCBs (in electrical oils, lubricants, adhesives, paints)
Insecticides:
- Atrazine (weed killer)
- DDT (dichlorodiphenyltrichloroethane) (insecticide, banned)
- Dichlorodiphenyldichloroethylene (one of the breakdown products of DDT)
- Dieldrin (insecticide)
- Endosulfan (insecticide)
- Heptachlor (insecticide)
- Lindane / hexachlorocyclohexane (insecticide, used to treat lice and scabies)
- Methoxychlor (insecticide)
- Fenthion
- Nonylphenol and derivatives (industrial surfactants; emulsifiers for emulsion polymerization; laboratory detergents; pesticides)
Other:
- Propyl gallate
- Chlorine and chlorine by-products
- Ethinylestradiol (combined oral contraceptive pill)
- Metalloestrogens (a class of inorganic xenoestrogens)
- Alkylphenol (surfactant used in cleaning detergents
- Triclosan
- Zeranol is currently used as an anabolic growth promoter for livestock in the US and Canada.
Xenoestrogens mechanism of actions
Xenoestrogens can affect the endocrine system at every level. First, they can disrupt the action of the enzymes involved in steroidogenesis. For example, a perturbation of aromatase activity can modify the estrogen/androgen balance and thus alter the development or the function of reproductive organs, as was observed with the tributyltin and some other pesticides 17. Other enzymes of the steroidogenesis can be impacted (mostly inhibited), as can the enzymes involved in metabolism of estrogens (Figure 3a). For instance, some PCB metabolites inhibit sulfotransferase, resulting in an increase of circulating estradiol rates 18. The transport of hormones can also be used as the target of certain compounds capable of interacting with the binding sites of SHBG (sex hormone binding globulin), thus competing with endogenous estrogens (Figure 3b) 19.
Figure 3. Xenoestrogens mechanism of actions
Footnote: Examples of interaction between xenoestrogens and estrogen signaling pathways. (a) Some xenoestrogens, such as tributyltins, can inhibit aromatase, the enzyme responsible for the conversion of androgens in estrogens, resulting in the perturbation of the androgen/estrogen balance. (b) Other groups of compounds can interfere with estrogenic signaling by competing with natural estrogens for binding to sex hormone binding globulin (SHBG), resulting in defects in 17𝛽-estradiol (E2) plasma transport. 20 Interaction between polycyclic aromatic hydrocarbons (PAHs) and estrogen response element-dependent 17𝛽-estradiol (E2)-target gene transcription. (c) Some PAH metabolites can bind estrogen receptor (ER), resulting in the recruitment of estrogen receptors at the estrogen response element and, subsequently, in the recruitment of coregulators that modulate the expression of 17𝛽-estradiol (E2) target genes. (d) Some polycyclic aromatic hydrocarbon metabolites or dioxin are also capable of binding to the aryl hydrocarbon receptor (AhR), resulting in heterodimerization with aryl hydrocarbon nuclear translocator (Arnt). This transcriptionally active complex can then interact with ligand-unbound estrogen receptor at the estrogen response element (ERE) site and modulate 17𝛽-estradiol (E2) target gene expression.
[Source 13 ]The most studied mode of actions of xenoestrogens is focusing the ability of these chemicals to bind and activate the estrogen receptors in target tissues 21. However, it is of note that the
two estrogen receptors mediate distinct biological effects in many tissues such as the mammary glands, bone, brain, and vascular system in both males and females. Therefore, because ER𝛼 and ER𝛽 show partially different tissue distribution and distinct physiological functions, xenoestrogens could display agonist or antagonist activity in a tissue-selective manner or during development. Considering the significant differences between estrogen receptor subtypes in structural features and relative ligand binding affinity, xenoestrogens can induce distinct conformational changes in the tertiary structure of the estrogen receptors, affecting the recruitment of cofactors differently. These interactions between estrogen receptors and coactivators/corepressors are critical steps in estrogen receptor-mediated transcriptional regulation and consequently the modulation of the expression of estrogen receptor-target genes. For example, the phytoestrogen genistein exhibits an affinity for ER𝛽 that is 20-fold superior to its affinity for ER𝛼 22. Moreover, the genistein effect is often tissue specific because it depends on numerous factors such as the expression of specific cofactors, the ER𝛼/ER𝛽 ratio, and the level of expression of certain intracellular kinases, including cytoplasmic tyrosine kinases. Genistein has been reported to have proliferative and antiproliferative effects in cancer cells 23.
Xenoestrogens generally act in 100–1000 folds greater concentrations than estradiol but can have additive or synergic effects with endogenous estradiol or when they are present in combination 11. Furthermore, the ability of some xenoestrogens to act as agonists in certain tissues and as antagonists in the others leads to the development and use of selective estrogen receptor modulators (SERMs), in particular for antihormonal treatments, such as tamoxifen and raloxifene.
Some xenoestrogens can also affect the estrogen receptor nongenomic pathways and induce an endocrine disruption 24. For instance, a recent study performed on structurally different xenoestrogens showed that at high concentrations, bisphenol A (BPA) and diethylstilbestrol (DES) are able to activate estrogen receptors via the activation of MAPK and PI3K in breast cancer cells.
In addition, the activation of PKC by some xenoestrogens has been observed 25. Interestingly, PKC has been reported to modulate ER𝛼 transcriptional activity 26. Therefore, synergic or additive effects between these pathways to combine the activation of estrogen receptor signaling could be envisaged.
Although the mechanistic studies on the interaction between dioxin and estrogen produced conflicting results, several studies reported that the ligands of AhR, such as polycyclic aromatic hydrocarbons (PAHs), can also affect estrogenic signaling in mammary or uterine cells (see Figure 3c). Ohtake et al. 27 showed that an AhR agonist, methylcholanthrene (3MC), is able to activate a reporter gene containing an estrogen response element (ERE) without affecting the expression level of estrogen receptor. However, when this promoter is activated by estradiol, methylcholanthrene (3MC) has an antagonistic effect. Coimmunoprecipitation assays showed that these functional interactions are correlated with the physical interaction between aryl hydrocarbon receptor (AhR) and estrogen receptor. The proposed model (Figure 3d) suggests that 3MC activates AhR, which dimerizes with aryl hydrocarbon nuclear translocator (Arnt). The AhR/ARNT heterodimer can directly associate with estrogen receptor that is not ligand bound to activate estrogen-sensitive gene transcription by recruiting the coactivator p300 27. This model is consolidated by in vivo experiments performed in the mouse. In fact, the proliferative effect of 3MC on the uterus is observed in ovariectomized mice, but not in aryl hydrocarbon receptor (AhR) knockout mice. These studies suggest an original mechanism of activation of ER𝛼 in the absence of estradiol because ligand-activated AhR is able to cooperate with the estrogen receptor that is not ligand bound to activate transcription. However, some metabolites of AhR ligands, including 3MC, could also behave as partial agonist on estrogen signaling pathways by direct interaction with ER𝛼 (Figure 3a).
Several mechanisms have also been proposed to describe the antiestrogenicity of AhR ligands 28. By binding to aryl hydrocarbon receptor (AhR), these compounds could interferewithtranscriptionally active estrogen receptor/SP1 or estrogen receptor/AP-1 complexes 29. They can also inhibit the binding of estrogen receptor to estrogen response element (ERE) sites by direct association with ER𝛼 27. The antiestrogenic effects of dioxins could also be mediated by the reduction of ER𝛼 protein level through activation of the proteasome 30. However, AhR-mediated degradation rates may vary according to the specific cellular context 28. Therefore, these interactions should be taken into account in the interpretations of studies that investigate the estrogenic effects of AhR ligands,particularly in mixtures.
Similarly, both the potent estrogenic and antiestrogenic effects of the heavy metal cadmium (Cd) have been reported in vitro in mammary cell lines, recombinant yeast assays, or fish hepatocyte cultures and in vivo in the rodent uterus 31. Although the precise mechanisms underlying the effects of heavy metal cadmium (Cd) as an endocrine disruptor remain unclear 32, two different mechanistic explanations were suggested. Cadmium (Cd) could directly interact with the ligand-binding domain of ER𝛼, inducing a conformational change in ligand-binding domain that favors the interaction between helix 12 of ER𝛼 with transcriptional coactivators 33. Other studies suggested that the interaction of the heavy metal cadmium (Cd) with the ligand-binding domain of ER𝛼 induces conformational changes in the DNA-binding domain which could inactivate the DNA binding activity of the receptor, reducing transactivation 31. However, cadmium is not the sole heavy metal able to interfere with estrogen signaling pathways, even if the precise modes of actions of these metalloestrogens are largely misunderstood 34.
Because endocrine disrupting chemical can also modulate hormonal signaling indirectly via their metabolites, endocrine disrupting chemical metabolism should be taken into account in the evaluation and identification of their mechanisms of action. For instance, the insecticide DDT and its metabolite dichlorodiphenyldichloroethylene (DDE) were characterized as weak estrogens in the environment and were suspected to affect reproduction function in several animal species 35. More recently, dichlorodiphenyldichloroethylene, which is highly lipophilic and resistant to biodegradation, was identified as the compound that induced the feminization of alligators (i.e., micropenis and various abnormalities of the testes) from Lake Apopka. These effects are likely mediated by the inhibition of androgen signaling during the critical developmental window 36. Although dichlorodiphenyldichloroethylene shows low affinity to estrogen receptors, it is capable of binding to androgen receptor and repressing the transcriptional activity of this receptor. This antiandrogenic action of dichlorodiphenyldichloroethylene shown in different cell-based assays could clearly cause abnormalities in the steroidogenic cells of rat testis and could disturb the development and function of fetal testis 37.
References- Anjum, Dr. Fakhsheena & Razvi, Nighat & Ghayas, Sana & Naeem, auj & Nisar, Ayesha. (2019). KNOWLEDGE AND ATTITUDE TOWARDS XENOESTROGENS. WORLD JOURNAL OF PHARMACY AND PHARMACEUTICAL SCIENCES. 8. 150-160. 10.20959/wjpps20198-14406.
- Co-evolution of steroidogenic and steroid-inactivating enzymes and adrenal and sex steroid receptors. Baker ME. Mol Cell Endocrinol. 2004 Feb 27; 215(1-2):55-62.
- Roy JR, Chakraborty S, Chakraborty TR. Estrogen-like endocrine disrupting chemicals affecting puberty in humans–a review. Medical Science Monitor, May 29, 2009; 15(6): RA137-45.
- Fernandez SV, Russo J. Estrogen and xenoestrogens in breast cancer. Toxicologic pathology, Jan, 2010; 38(1): 110-22.
- Darbre PD. Environmental oestrogens, cosmetics and breast cancer. Best practice & research clinical endocrinology & metabolism, Mar 1, 2006; 20(1): 121-43.
- J.M. Harrington, G. F. Stein, R. O. Rivera, and A. V. deMorales, “The occupational hazards of formulating oral contraceptives. A survey of plant employees,” Archives of Environmental Health, vol. 33, no. 1, pp. 12–15, 1978.
- G. H. Degen and H. M. Bolt, “Endocrine disruptors: update on xenoestrogens,” International Archives of Occupational and Environmental Health, vol. 73, no. 7, pp. 433–441, 2000.
- R. R. Newbold, “Prenatal exposure to diethylstilbestrol (DES),” Fertility and Sterility, vol. 89, no. 2, pp. e55–e56, 2008.
- L. Multigner, J. R. Ndong, A. Giusti et al., “Chlordecone exposure and risk of prostate cancer,” Journal of Clinical Oncology, vol. 28, no. 21, pp. 3457–3462, 2010.
- Z. H. Liu, Y. Kanjo, and S. Mizutani, “A review of phytoestrogens: their occurrence and fate in the environment,” Water Research, vol. 44, no. 2, pp. 567–577, 2010.
- A. Kortenkamp and R. Altenburger, “Synergisms withmixtures of xenoestrogens: a reevaluation using the method of isoboles,” Science of the Total Environment, vol. 221, no. 1, pp. 59–73, 1998.
- D. W. Singleton and S. A. Khan, “Xenoestrogen exposure and mechanisms of endocrine disruption,” Frontiers in Bioscience, vol. 8, pp. s110–s118, 2003.
- Kerdivel, Gwenneg & Habauzit, Denis & Pakdel, Farzad. (2013). Assessment and Molecular Actions of Endocrine-Disrupting Chemicals That Interfere with Estrogen Receptor Pathways. International journal of endocrinology. 2013. 501851. 10.1155/2013/501851.
- Fucic A, Gamulin M, Ferencic Z, Katic J, von Krauss MK, Bartonova A, Merlo DF. Environmental exposure to xenoestrogens and oestrogen related cancers: reproductive system, breast, lung, kidney, pancreas, and brain. Environmental Health, Jun, 2012; 11(1): S8.
- Brody JG, Rudel RA. Environmental pollutants and breast cancer. Environmental health perspectives, Jun, 2003; 111(8): 1007.
- Weschler CJ. Changes in indoor pollutants since the 1950s. Atmospheric Environment, Jan 1, 2009; 43(1): 153-69.
- D. D. Heidrich, S. Steckelbroeck, and D. Klingmuller, “Inhibition of human cytochrome P450 aromatase activity by butyltins,” Steroids, vol. 66, no. 10, pp. 763–769, 2001.
- M. H. A. Kester, S. Bulduk, D. Tibboel et al., “Potent inhibition of estrogen sulfotransferase by hydroxylated PCB metabolites: a novel pathway explaining the estrogenic activity of PCB’s,” Endocrinology, vol. 141, no. 5, pp. 1897–1900, 2000.
- H. D´echaud, C. Ravard, F. Claustrat, A. B. de la Perri`ere, andM. Pugeat, “Xenoestrogen interaction with human sex hormonebinding globulin (hSHBG),” Steroids, vol. 64,no. 5, pp. 328–334, 1999.
- c) and (d
- L. Celik, J.D.D. Lund, and B. Schiøtt, “Exploring interactions of endocrine-disrupting compounds with different conformations of the human estrogen receptor 𝛼 ligand binding domain: a molecular docking study,” Chemical Research in Toxicology, vol. 21, no. 11, pp. 2195–2206, 2008.
- E. J. Routledge, R.White, M. G. Parker, and J. P. Sumpter, “Differential effects of xenoestrogens on coactivator recruitment by estrogen receptor (ER) 𝛼 and ER𝛽,” The Journal of Biological Chemistry, vol. 275, no. 46, pp. 35986–35993, 2000.
- S. Jagadeesh, S. Kyo, and P. P. Banerjee, “Genistein represses telomerase activity via both transcriptional and posttranslational mechanisms in human prostate cancer cells,” Cancer Research, vol. 66, no. 4, pp. 2107–2115, 2006.
- P. H. Watson, R. T. Pon, and R. P. C. Shiu, “Inhibition of cmyc expression by phosphorothioate antisense oligonucleotide identifies a critical role for c-myc in the growth of human breast cancer,” Cancer Research, vol. 51, no. 15, pp. 3996–4000, 1991.
- X. Li, S. Zhang, and S. Safe, “Activation of kinase pathways in MCF-7 cells by 17𝛽-estradiol and structurally diverse estrogenic compounds,” Journal of Steroid Biochemistry and Molecular Biology, vol. 98, no. 2-3, pp. 122–132, 2006.
- H. Cho and B. S. Katzenellenbogen, “Synergistic activation of estrogen receptor-mediated transcription by estradiol and protein kinase activators,” Molecular Endocrinology, vol. 7, no. 3, pp. 441–452, 1993.
- F. Ohtake, K. I. Takeyama, T. Matsumoto et al., “Modulation of oestrogen receptor signalling by association with the activated dioxin receptor,” Nature, vol. 423, no. 6939, pp. 545–550, 2003.
- S. Safe andM.Wormke, “Inhibitory aryl hydrocarbon receptorestrogen receptor 𝛼 cross-talk and mechanisms of action,” Chemical Research in Toxicology, vol. 16,no. 7, pp. 807–816, 2003.
- S. Khan, R. Barhoumi, R. Burghardt, S. Liu, K. Kim, and S. Safe, “Molecular mechanism of inhibitory aryl hydrocarbon receptor—estrogen receptor/Sp1 cross talk in breast cancer cells,” Molecular Endocrinology, vol. 20, no. 9, pp. 2199–2214, 2006.
- M.Wormke, M. Stoner, B. Saville et al., “The aryl hydrocarbon receptor mediates degradation of estrogen receptor 𝛼 through activation of proteasomes,” Molecular and Cellular Biology, vol. 23, no. 6, pp. 1843–1855, 2003.
- E. Silva, M. J. Lopez-Espinosa, J. M. Molina-Molina, M. Fern´andez, N. Olea, and A. Kortenkamp, “Lack of activity of cadmium in in vitro estrogenicity assays,” Toxicology and Applied Pharmacology, vol. 216, pp. 20–28, 2006.
- M. Takiguchi and S. Yoshihara, “New aspects of cadmium as endocrine disruptor,” Environmental Sciences, vol. 13, no. 2, pp. 107–116, 2006.
- A. Stoica, B. S. Katzenellenbogen, andM.B.Martin, “Activation of estrogen receptor-𝛼 by the heavymetal cadmium,”Molecular Endocrinology, vol. 14, no. 4, pp. 545–553, 2000.
- C. Byrne, S.D.Divekar,G. B. Storchan, D. A. Parodi, and M. B. Martin, “Metals and breast cancer,” Journal of Mammary Gland Biology and Neoplasia, vol. 18, pp. 63–73, 2013.
- L. J. Guillette Jr., T. S. Gross, G. R. Masson, J. M. Matter, H. F. Percival, and A. R. Woodward, “Developmental abnormalities of the gonad and abnormal sex hormone concentrations in juvenile alligators from contaminated and control lakes in Florida,” Environmental Health Perspectives, vol. 102, no. 8, pp. 680–688, 1994.
- W.R.Kelce, C.R. Stone, S. C. Laws, L. E.Gray, J. A.Kemppainen, and E. M. Wilson, “Persistent DDT metabolite p,p’-DDE is a potent androgen receptor antagonist,” Nature, vol. 375, no. 6532, pp. 581–585, 1995.
- A. Thiel, S. Guth, S. B¨ohm, and G. Eisenbrand, “Dicofol degradation to p,p’-dichlorobenzophenone—a potential antiandrogen,” Toxicology, vol. 282, no. 3, pp. 88–93, 2011.