Human brain
The human brain is a component of the central nervous system. The human brain is roughly the size of two clenched fists and weighs about 1.6 kg (3.5 lb) in men and 1.45 kg in women 1. The difference between the sexes is proportional to body size, not intelligence. The organs of the central nervous system (CNS) can be divided into two groups, the brain and the spinal cord.
Anatomists conceptually divide the brain into four major parts—
- The Cerebrum.
- The Diencephalon (thalamus, hypothalamus, and epithalamus).
- The Cerebellum.
- The Brainstem.
Cerebrum
The cerebrum is the large, outer part of the brain. The cerebrum is about 83% of the brain’s volume and consists of a pair of half globes called the cerebral hemispheres. Each hemisphere is marked by thick folds called gyri (singular, gyrus) separated by shallow grooves called sulci (singular, sulcus). A very deep median groove, the longitudinal fissure, separates the right and left hemispheres from each other. At the bottom of this fissure, the hemispheres are connected by a thick bundle of nerve fibers called the corpus callosum—a prominent landmark for anatomical description with a distinctive C shape in sagittal section.
The cerebral hemispheres control reasoning, thought, emotion, and language. It is also responsible for planned (voluntary) muscle movements (throwing a ball, walking, chewing, etc.) and for taking in and interpreting sensory information such as vision, hearing, smell, touch, and pain.
Figure 1. Human brain
Cerebellum
The cerebellum occupies the posterior cranial fossa inferior to the cerebrum, separated from it by the transverse cerebral fissure. It is also marked by fissures, sulci, and gyri (called folia in the cerebellum). The cerebellum is the second-largest region of the brain, constituting about 10% of its volume but containing over 50% of its neurons.
The cerebellum lies under the cerebrum at the back part of the brain. It helps coordinate movement. Tumors of the cerebellum can cause problems with coordination in walking, trouble with precise movements of hands, arms, feet, and legs, problems swallowing or synchronizing eye movements, and changes in speech rhythm.
Brain stem
The brain stem is the lower part of the brain that connects to the spinal cord. It contains bundles of very long nerve fibers that carry signals controlling muscles and sensation or feeling between the cerebrum and the rest the body. Special centers in the brain stem also help control breathing and the beating of the heart. Also, most cranial nerves (see Figures 1 and 2) start in the brain stem.
The brainstem is defined all of the brain except the cerebrum and cerebellum. Its major components, from rostral (front towards the nose) to caudal (back end), are the midbrain, pons, and medulla oblongata. In a living person, the brainstem is oriented like a vertical stalk with the cerebrum perched on top like a mushroom cap. Postmortem changes give it a more oblique angle in the cadaver and consequently, in many medical illustrations. Towards the back end, the brainstem ends at the foramen magnum of the skull, and the central nervous system (CNS) continues below this as the spinal cord.
Figure 2. Medial aspect of the human brain
The brain, like the spinal cord, is composed of gray and white matter. Gray matter—the seat of the neurosomas, dendrites, and synapses—forms a surface layer called the cortex over the cerebrum and cerebellum, and deeper masses called nuclei surrounded by white matter. White matter lies deep to the cortical gray matter in most of the brain, opposite from the relationship of gray and white matter in the spinal cord. As in the spinal cord, white matter is composed of tracts, or bundles of axons, which here connect one part of the brain to another and to the spinal cord.
Parts of the brain and their functions
During fetal development the brain can be divided into five continuous parts (see Figure 3). From top to bottom they are :
- Prosencephalon (the embryonic forebrain):
- The telencephalon (cerebrum) becomes the large cerebral hemispheres. The surface of these hemispheres consists of elevations (gyri) and depressions (sulci) and the hemispheres are partially separated by a deep longitudinal fissure. The cerebrum fills the area of the skull above the tentorium cerebelli and is subdivided into lobes based on position.
- The diencephalon, which is hidden from view in the adult brain by the cerebral hemispheres, consists of the thalamus, hypothalamus, and epithalamus and third ventricle.
- Mesencephalon or the midbrain, gives rise to the midbrain and aqueduct of the midbrain (cerebral aqueduct).
- Rhombencephalon (the hindbrain):
- The metencephalon becomes the pons, cerebellum, and upper part of the fourth ventricle.
- The myelencephalon forms the medulla oblongata and lower part of the fourth ventricle.
Figure 3. Parts of the brain
Note: (a) The primary vesicles at 4 weeks (fetus). (b) The secondary vesicles at 5 weeks (fetus). (c) The fully developed brain, color-coded to relate its structures to the secondary embryonic vesicles.
The Cerebrum
The cerebrum, the most upper and outer portion of the brain, is made up of two cerebral hemispheres and together these hemispheres account for 83% of total brain mass. They so dominate the brain that many people mistakenly use the word brain when referring specifically to the cerebrum. The cerebral hemispheres cover the diencephalon and the brain stem in much the same way a mushroom cap covers the top of its stalk.
The various fissures evident on and around the cerebral hemispheres separate the major portions of the brain from one another. The transverse cerebral fissure separates the cerebral hemispheres from the cerebellum inferiorly, whereas the median longitudinal fissure separates the right and left cerebral hemispheres from each other. The cerebrum is composed of a superficial cerebral cortex of gray matter, the cerebral white matter internal to it, and the deep gray matter of the cerebrum within the white matter.
Both cerebral hemispheres participate in basic functions, such as receiving and analyzing sensory impulses, controlling skeletal muscles, and storing memory. However, in most individuals, one side of the cerebrum is the dominant hemisphere, controlling the ability to use and understand language. In most people the left hemisphere is dominant for the language-related activities of speech, writing, and reading, and for complex intellectual functions requiring verbal, analytical,
and computational skills. In others, the right hemisphere is dominant for language-related abilities, or the hemispheres are equally dominant. Broca’s area in the dominant hemisphere controls the muscles that function in speaking.
In addition to carrying on basic functions, the nondominant hemisphere specializes in nonverbal functions, such as motor tasks that require orientation of the body in space, understanding and interpreting musical patterns, and nonverbal visual experiences. The nondominant hemisphere also controls emotional and intuitive thinking. Nerve fibers of the corpus callosum, which connect the cerebral hemispheres, allow the dominant hemisphere to control the motor cortex of the nondominant hemisphere. These fibers also transfer sensory information reaching the nondominant hemisphere to the dominant one, where the information can be used in decision making.
Lobes of the Cerebral Cortex
There are many shallow grooves on the surface of the cerebral hemispheres called sulci (singular: sulcus, “furrow”, “throughs”). Between the sulci are twisted ridges of brain tissue called gyri (singular: gyrus, “twister”). The more prominent gyri and sulci are similar in all people and are important anatomical landmarks. Some of the deeper sulci divide each cerebral hemisphere into five major lobes:
- The Frontal lobe,
- The Parietal lobe,
- The Occipital lobe,
- The Temporal lobe,
- The Insula lobe.
Most of these lobes are named for the skull bones overlying them.
Figure 4. Cerebrum of the brain
Frontal lobe of brain
The frontal lobe is located deep to the frontal bone and fills the anterior cranial fossa. It extends posteriorly to the central sulcus, which separates the frontal lobe from the parietal lobe. The precentral gyrus containing the primary motor cortex lies just anterior to the central sulcus.
The frontal lobe contains functional areas that plan, initiate, and enact motor movement including eye movement and speech production. The most anterior region of the frontal cortex performs higher-order cognitive functions, such as thinking, planning, decision making, working memory, and other executive functions.
Parietal lobe of brain
The parietal lobe, deep to the parietal bones, extends posteriorly from the central sulcus to the parieto-occipital sulcus. The lateral sulcus forms its inferior boundary. The postcentral gyrus, just posterior to the central sulcus, contains the primary somatosensory cortex. The parietal lobe processes sensory stimuli allowing (1) conscious awarness of general somatic sensation; (2) spacial awarness of objects, sounds and body parts; and (3) understanding of speech.
Occipital lobe of brain
The occipital lobe lies deep to the occipital bone and forms the most posterior portion of the cerebrum. It is separated from the parietal lobe by the parieto-occipital sulcus on the medial surface of the hemiphere. The occipital lobe contains the visual cortex.
Temporal lobe of brain
The temporal lobe, on the lateral side of the hemisphere, lies in the middle cranial fossa deep to the temporal bone. It is separated from the overlying parietal and frontal lobes by the deep lateral sulcus. The temporal lobe contains the auditory cortex and the olfactory cortex. It also functions in the recognition of objects, words, and faces; in language comprehension; and in emotional response and memory.
Insula lobe of brain
The insula (“island”) is buried deep within the lateral sulcus and forms part of its floor. The insula is covered by parts of the temporal, parietal, and frontal lobes. The visceral sensory cortex for taste and general visceral sensations are in the insula.
Cerebral White Matter
The cerebral white matter consists primarily of myelinated axons in three types of tracts:
- Association tracts contain axons that conduct nerve impulses between gyri in the same hemisphere.
- Commissural tracts contain axons that conduct nerve impulses from gyri in one cerebral hemisphere to corresponding gyri in the other cerebral hemisphere. Three important groups of commissural tracts are the corpus callosum (the largest fiber bundle in the brain, containing about 300 million fibers), anterior commissure, and posterior commissure.
- Projection tracts contain axons that conduct nerve impulses from the cerebrum to lower parts of the central nervous system (thalamus, brainstem or spinal cord) or from lower parts of the central nervous system to the cerebrum. An example is the internal capsule, a thick band of white matter that contains both ascending and descending axons.
Cerebral Gray Matter (Functional Areas of the Cerebral Cortex)
The cerebral cortex is composed of gray matter because it contains neuron cell bodies, dendrites, and very short unmyelinated axons but no fiber tracts. Even though the cerebral cortex is only 2–4 mm thick, its many gyri and sulci triple its surface area to approxmately 2500 cm2, about the size of a large desk calendar, and it accounts for about 40% of the total mass of the brain.
The cerebral cortex contains 14 to 16 billions of neurons and about 90% of the human cerebral cortex is a six-layered tissue called neocortex because of its relatively recent evolutionary origin. The six layers of neocortex, vary from one part of the cerebrum to another in relative thickness, cellular composition, synaptic connections, size of the neurons, and destination of their axons. Layer IV is thickest in sensory regions and layer V in motor regions, for example. All axons that leave the cortex and enter the white matter arise from layers III, V, and VI.
Figure 5. Gray matter of the neocortex
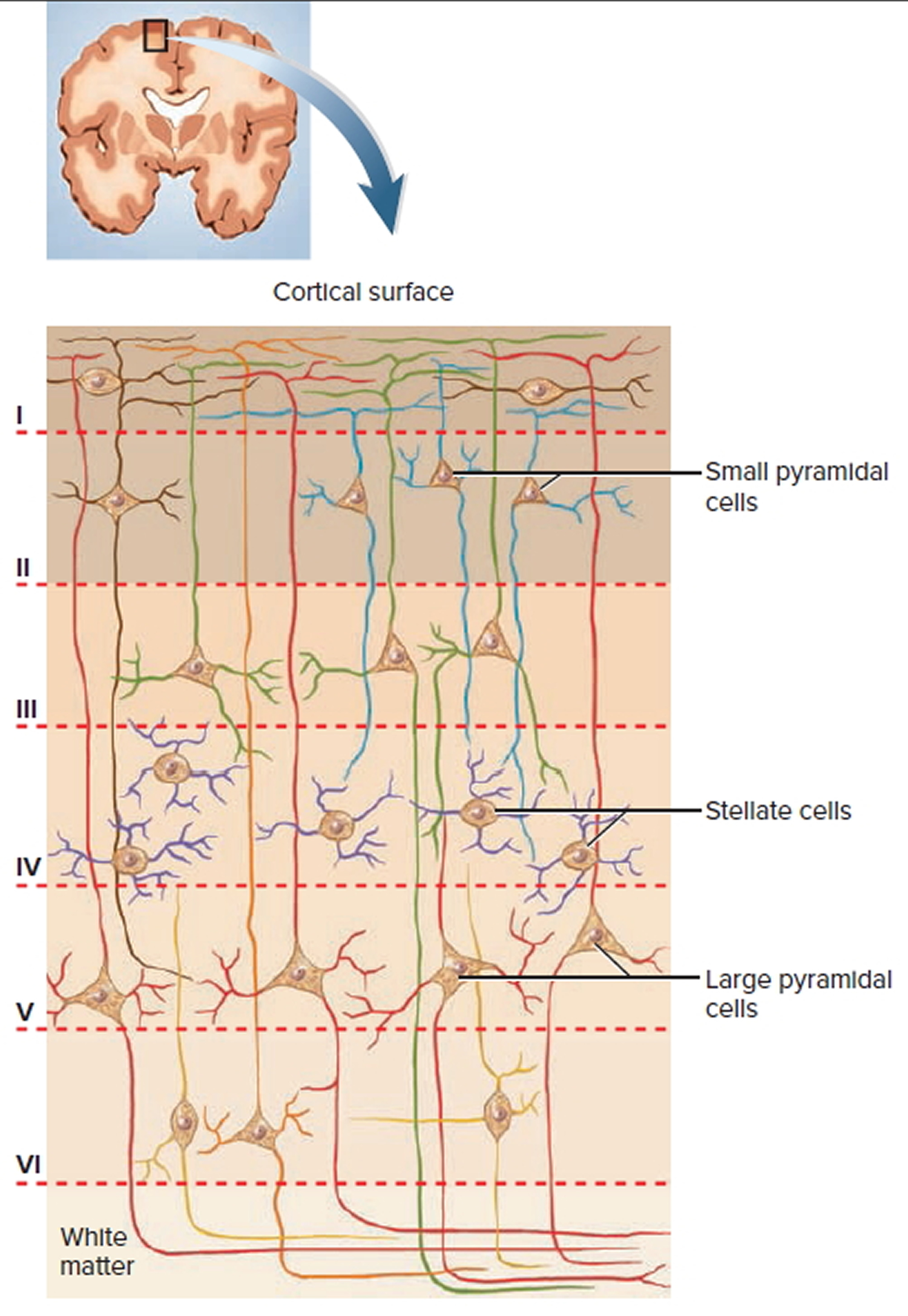
The cerebral cortex possesses two principal types of neurons called stellate cells and pyramidal cells. Stellate cells have spheroidal somas with short axons and dendrites projecting in all directions. They are concerned largely with receiving sensory input and processing information on a local level. Pyramidal cells are tall and conical. Their apex points toward the brain surface and has a thick dendrite with many branches and small, knobby dendritic spines. The base gives rise to horizontally oriented dendrites and an axon that passes into the white matter. Pyramidal cells include the output neurons of the cerebrum—the only cerebral neurons whose fibers leave the cortex and connect with other parts of the CNS. Pyramidal cell axons have collaterals that synapse with other neurons in the cortex or in deeper regions of the brain.
In 1909, a German neurologist, Korbinian Brodmann, mapped the cerebral cortex into 47 structural areas based on subtle variations in the thickness of the six layers. In the 21st century with the advent of functional neuroimaging techniques—PET (positron emission tomography) and fMRI (functional magnetic resonance imaging)—these techniques reveal areas of maximal metabolic activity and blood flow to the brain, which are presumed to be areas participating in the mental task being performed. A fMRI obtained during a variety of tasks indicates areas of increased blood flow, as shown by the red-yellow color, in the regions of the cerebral cortex associated with each task.
Three general kinds of functional areas are recognized in the cerebral cortex:
- Sensory areas, which allow conscious awareness of sensation;
- Association areas, which integrate diverse information to enable purposeful action; and
- Motor areas, which control voluntary motor functions.
Figure 6. Functional areas of the cerebral cortex
There is a sensory area for each of the major senses. Each region is called a primary sensory cortex. Each primary sensory cortex has association areas linked to it that process the sensory information. These areas are sensory association areas. Other association areas receive and integrate input from multiple regions of the cerebral cortex. These regions are called multimodal association areas. Finally, the regions of the cortex that plan and initiate voluntary motor functions are called the motor areas. Information is processed through these regions of the cerebral cortex in the following hierarchical manner.
- Sensory information is received by the primary sensory cortex, and the arrival of this information results in awareness of the sensation.
- The information is relayed to the sensory association area that gives meaning to the sensory input.
- The multimodal association areas receive input in parallel from multiple sensory association areas, integrating all of the sensory input to create a complete understanding of the sensory information. These regions also integrate sensory input with past experience and develop a motor response.
- The motor plan is enacted by the motor cortex.
Figure 7. Primary motor cortex and somatosensory cortex of the cerebrum
Basal Nuclei
Deep within each cerebral hemisphere are three nuclei (masses of gray matter) that are collectively termed the basal nuclei. Historically, these nuclei have been called the basal ganglia. Two of the basal nuclei lie side by side, just lateral to the thalamus. They are the globus pallidus, which is closer to the thalamus, and the putamen, which is closer to the cerebral cortex. Together, the globus pallidus and putamen are referred to as the lentiform nucleus. The third of the basal nuclei
is the caudate nucleus, which has a large “head” connected to a smaller “tail” by a long, comma-shaped “body.”
Together, the lentiform and caudate nuclei are known as the corpus striatum. The term corpus striatum refers to the striated (striped) appearance of the internal capsule as it passes among the basal nuclei. Nearby structures that are functionally linked to the basal nuclei are the substantia nigra of the midbrain and the subthalamic nuclei of the diencephalon. Axons from the substantia nigra terminate in the caudate nucleus and putamen. The subthalamic nuclei interconnect
with the globus pallidus.
Figure 8. Basal nuclei of human brain
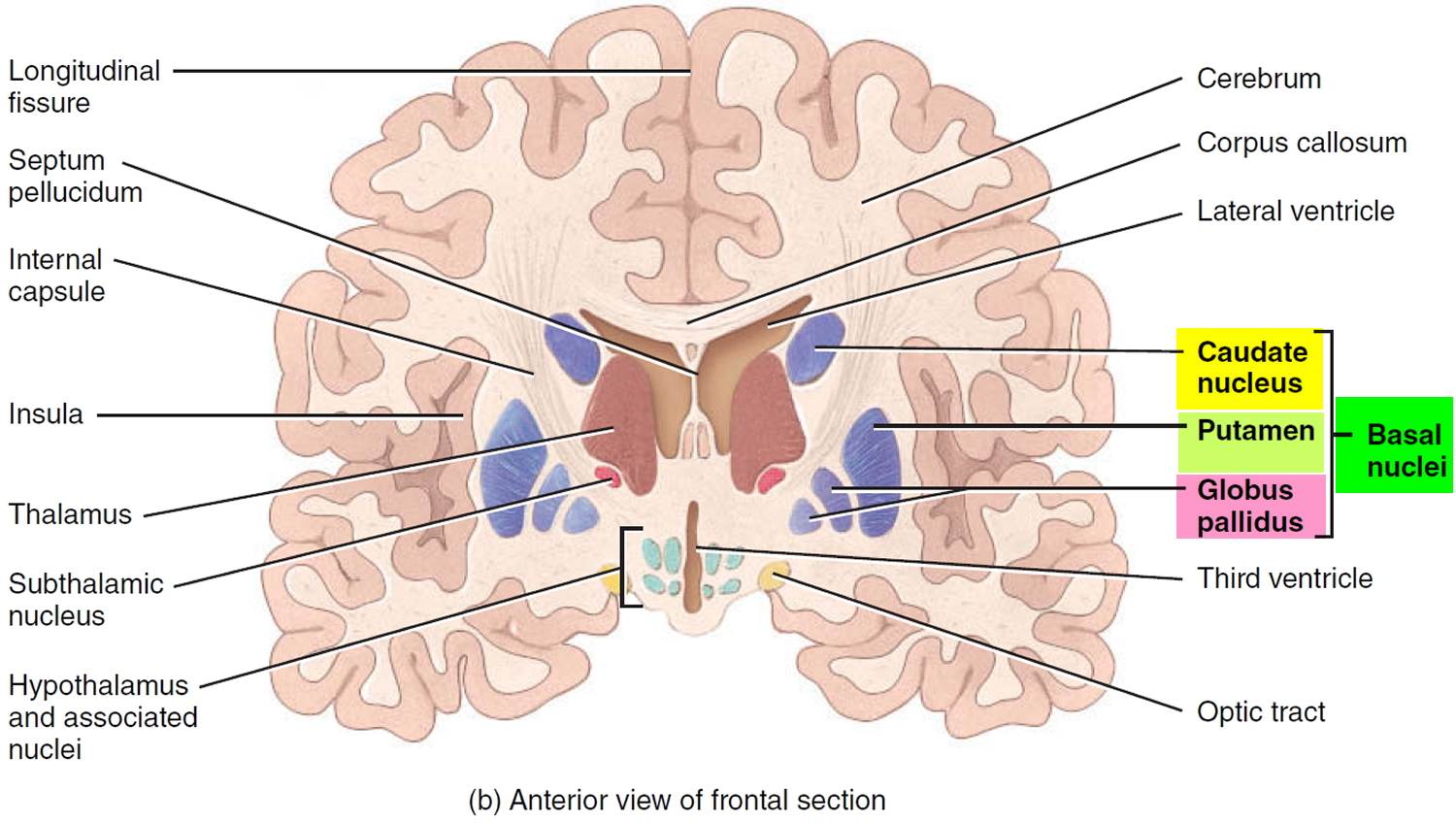
The claustrum is a thin sheet of gray matter situated lateral to the putamen. It is considered by some to be a subdivision of the basal nuclei. The function of the claustrum in humans has not been clearly defined, but it may be involved in visual attention.
The basal nuclei receive input from the cerebral cortex and provide output to motor parts of the cortex via the medial and ventral group nuclei of the thalamus. In addition, the basal nuclei have extensive connections with one another. A major function of the basal nuclei is to help regulate initiation and termination of movements. Activity of neurons in the putamen precedes or anticipates body movements; activity of neurons in the caudate nucleus occurs prior to eye movements.
The globus pallidus helps regulate the muscle tone required for specific body movements. The basal nuclei also control subconscious contractions of skeletal muscles. Examples include automatic arm swings while walking and true laughter in response to a joke. In addition to influencing motor functions, the basal nuclei have other roles. They help initiate and terminate some cognitive processes, attention, memory, and planning, and may act with the limbic system to
regulate emotional behaviors. Disorders such as Parkinson’s disease, obsessive–compulsive disorder, schizophrenia, and chronic anxiety are thought to involve dysfunction of circuits between the basal nuclei and the limbic system.
The Diencephalon
Deep in the core area of the brain, just above the top of the brainstem, is the diencephalon that consists largely of three paired structures—the thalamus, the hypothalamus and the epithalamus. The diencephalon are structures that have a great deal to do with perception, movement, and the body’s vital functions.
Figure 9. Diencephalon of the human brain
The Thalamus
The egg-shaped thalamus is a paired structure that makes up 80% of the diencephalon and forms the superolateral walls of the third ventricle. Usually the right and left parts of the thalamus are joined by a small midline connection, the interthalamic adhesion (intermediate mass).
The thalamus consists of two oval masses that contain about a dozen major nuclei, each of which sends axons to a particular portion of the cerebral cortex (Figure 5). The thalamic masses contain nerve cell bodies that sort information from four of the senses—sight, hearing, taste, and touch—and relay it to the cerebral cortex. (Only the sense of smell sends signals directly to the cortex, bypassing the thalamus.) Sensations of pain, temperature, and pressure are also relayed through the thalamus, as are the nerve impulses from the cerebral hemispheres that initiate voluntary movement.
For example,
- The ventral posterolateral nuclei act as relay stations for the sensory information ascending to the primary sensory areas of the cerebral cortex.
- The medial geniculate body receives auditory input and links to the auditory cortex.
- The lateral geniculate body receives visual input and transmits to the visual cortex.
Sensory inputs are not the only type of information relayed through the thalamus. Every part of the brain that communicates with the cerebral cortex must relay its signals through a nucleus of the thalamus. The thalamus can therefore be thought of as the “gateway” to the cerebral cortex.
The thalamus not only relays information to the cerebral cortex but also processes the information as it passes through.
The thalamic nuclei organize and then either amplify or “tone down” the signals headed for the cerebral cortex. This is why, for example, you can focus on a conversation with a single person in large, noisy cafeteria.
Figure 10. Thalamus and Hypothalamus of the brain
The Hypothalamus
The hypothalamus (“below the thalamus”) is the inferior portion of the diencephalon. Projecting inferiorly from the hypothalamus is the pituitary gland (Figure 1) and the hypothalamus occupies approximately 2 per cent of the brain volume. The hypothalamus is situated in a strategic position at the crossroad of four systems, neurovegetative, neuroendocrine, limbic, and optic 2.
The hypothalamus forms the inferolateral walls of the third ventricle. On the underside of the brain, the hypothalamus lies between the optic chiasma (point of crossover of cranial nerves II, the optic nerves) and the posterior border of the mammillary bodies, rounded bumps that bulge from the hypothalamic floor (mammillary = “little breast”).
The hypothalamus, like the thalamus, contains about a dozen brain nuclei of gray matter. Despite its relatively small size (roughly that of a thumbnail or an almond), functionally, the hypothalamus is the main visceral control center of the body, regulating many activities of the visceral organs.
The hypothalamus is the main point of interaction for the body’s two physical control systems: the nervous system, which transmits information in the form of minute electrical impulses, and the endocrine system, which brings about changes of state through the release of chemical factors. It is the hypothalamus that first detects crucial changes in the body and responds by stimulating various glands and organs to release hormones.
Its functions include the following:
- Control of the autonomic nervous system. At the autonomic level, the hypothalamus stimulates smooth muscle (which lines the blood vessels, stomach, and intestines) and receives sensory impulses from these areas. Thus it controls the heart rate and blood pressure, the passage of food through the alimentary canal, the secretion from sweat glands and salivary glands and contraction of the bladder and many other visceral activities. The hypothalamus
exerts its control over visceral functions by relaying its instructions through the periaqueductal gray matter of the midbrain and the reticular formation of the brain stem, which then carry out those instructions.
- Regulation of body temperature. The body’s thermostat is in the hypothalamus. In the hypothalamus are neurons that monitor body temperature at the surface through nerve endings in the skin and other neurons that monitor the blood flowing through this part of the brain itself, as an indicator of core body temperature. The front part of the hypothalamus contains neurons that act to lower body temperature by relaxing smooth muscle in the blood vessels, which causes them to dilate and increases the rate of heat loss from the skin. Through its neurons associated with the sweat glands of the skin, the hypothalamus can also promote heat loss by increasing the rate of perspiration. In opposite conditions, when the body’s temperature falls below the (rather narrow) ideal range, a portion of the hypothalamus directs the contraction of blood vessels, slows the rate of heat loss, and causes the onset of shivering (which produces a small amount of heat). Hypothalamic centers also induce fever.
- Regulation of hunger and thirst sensations. The hypothalamus is the control center for the stimuli that underlie eating and drinking. By sensing the concentrations of nutrients and salts in the blood, certain hypothalamic neurons mediate feelings of hunger and thirst and thus aid in maintaining the proper concentrations of these substances. The sensations that you interpret as hunger arise partly from a degree of emptiness in the stomach and partly from a drop in the level of two substances: glucose circulating in the blood and a hormone that the intestine produces shortly after the intake of food. Receptors for this hormone gauge how far digestion has proceeded since the last meal. This system is not a simple “on” switch for hunger, however: another portion of the hypothalamus, when stimulated, actively inhibits eating by promoting a feeling of satiety. In experimental animals, damage to this portion of the brain is associated with continued excessive eating, eventually leading to obesity.
- Regulation of sleep-wake cycles. Acting with other brain regions, the hypothalamus helps regulate the complex phenomenon of sleep. The suprachiasmatic nucleus is the body’s biological clock. It generates the daily circadian rhythms and synchronizes these cycles in response to dark-light information sensed via the optic nerve. In response to such signals, the preoptic nucleus induces sleep. Electrical stimulation of a portion of the hypothalamus has been shown to induce sleep in experimental animals, although the mechanism by which this works is not yet known. Other hypothalamic nuclei near the mammillary body mediate arousal from sleep. Furthermore, the hypothalamus forms part of the reticular activating system, the physical basis for that hard-to-define state known as consciousness.
- Control of the endocrine system. The hypothalamus controls the secretion of hormones by the pituitary gland, which in turn influences the activity of many other endocrine organs.
- Control of emotional responses. The hypothalamus lies at the center of the emotional part of the brain, the limbic system. Regions involved in pleasure, rage, and fear are located in the hypothalamus. The hypothalamus is the brain’s intermediary for translating emotion into physical response. When strong feelings (rage, fear, pleasure, excitement) are generated in the mind, whether by external stimuli or by the action of thoughts, the cerebral cortex transmits impulses to the hypothalamus; the hypothalamus may then send signals for physiological changes through the autonomic nervous system and through the release of hormones from the pituitary. Physical signs of fear or excitement, such as a racing heartbeat, shallow breathing, and perhaps a clenched “gut feeling,” all originate here.
- Control of motivational behavior. The hypothalamus controls behavior that is rewarding. For example, the hypothalamus influences your motivation for feeding, thereby determining how much you eat, and also influences sex drive and sexual behavior.
- Formation of memory. The brain nucleus in the mammillary body receives many inputs from the major memory processing structure of the cerebrum, the hippocampal formation.
Lesions of the hypothalamus cause disorders in visceral functions and in emotions. Thus, injuries to the hypothalamus can result in severe weight loss or obesity, sleep disturbances, dehydration, and a broad range of emotional disorders.
In all, the hypothalamus is a richly complex cubic centimeter of vital connections, which will continue to reward close study for some time to come. Because of its unique position as a midpost between thought and feeling and between conscious act and autonomic function, a thorough understanding of its workings should tell us much about the earliest history and development of the human animal.
The Epithalamus
The epithalamus, the third and most dorsal (back end) part of the diencephalon, forms part of the roof of the third ventricle. It consists of one tiny group of brain nuclei and a small, unpaired knob called the pineal gland. This gland, which derives from ependymal glial cells, is a hormone-secreting organ. Under the influence of the hypothalamus, the pineal gland secretes the hormone melatonin, which signals the body to prepare for the nighttime stage of the sleep-wake cycle.
The Limbic System
The limbic system is sometimes called the “emotional brain” because it plays a primary role in a range of emotions, including pain, pleasure, docility, affection, and anger. It also is involved in olfaction (smell), learning and memory.
Together with parts of the cerebrum, the limbic system also functions in memory; damage to the limbic system causes memory impairment. One portion of the limbic system, the hippocampus, is seemingly unique among structures of the central nervous system—it has cells reported to be capable of mitosis. Thus, the portion of the brain that is responsible for some aspects of memory may develop new neurons, even in the elderly.
The limbic system is a ring of cortex on the medial side of each hemisphere, encircling the corpus callosum and thalamus. The main components of the limbic system are as follows:
- The so-called limbic lobe is a rim of cerebral cortex on the medial surface of each hemisphere. It includes the cingulate gyrus, which lies above the corpus callosum, and the parahippocampal gyrus, which is in the temporal lobe below. The hippocampus is a portion of the parahippocampal gyrus that extends into the floor of the lateral ventricle.
- The dentate gyrus lies between the hippocampus and parahippocampal gyrus.
- The amygdala is composed of several groups of neurons located close to the tail of the caudate nucleus.
- The septal nuclei are located within the septal area formed by the regions under the corpus callosum and the paraterminal gyrus (a cerebral gyrus).
- The mammillary bodies of the hypothalamus are two round masses close to the midline near the cerebral peduncles.
- Two nuclei of the thalamus, the anterior nucleus and the medial nucleus, participate in limbic circuits.
- The olfactory bulbs are flattened bodies of the olfactory pathway that rest on the cribriform plate.
- The fornix, stria terminalis, stria medullaris, medial forebrain bundle, and mammillothalamic tract are linked by bundles of interconnecting myelinated axons.
Its most anatomically prominent components are the cingulate gyrus, which arches over the top of the corpus callosum in the frontal and parietal lobes; the hippocampus in the medial temporal lobe; and the amygdala immediately rostral to the hippocampus, also in the temporal lobe. There are still differences of opinion on what structures to consider as parts of the limbic system, but these three are agreed upon.
Other components include the mammillary bodies and other hypothalamic nuclei, some thalamic nuclei, parts of the basal nuclei, and parts of the frontal lobe called prefrontal and orbitofrontal cortex. Limbic system components are interconnected through a complex loop of fiber tracts allowing for somewhat circular patterns of feedback among its nuclei and cortical neurons. All of these structures are bilaterally paired; there is a limbic system in each cerebral hemisphere.
The limbic system was long thought to be associated with smell because of its close association with olfactory pathways, but beginning in the early 1900s and continuing even now, experiments have abundantly demonstrated more significant roles in emotion and memory. Most limbic system structures have centers for both gratification and aversion. Stimulation of a gratification center produces a sense of pleasure or reward; stimulation of an aversion center produces unpleasant sensations such as fear or sorrow. Gratification centers dominate some limbic structures, such as the nucleus accumbens (not illustrated), while aversion centers dominate others such as the amygdala. The roles of the amygdala in emotion and the hippocampus in memory.
Figure 11. Limbic system
The Cerebellum
The cerebellum is the largest part of the hindbrain and second largest part of the brain as a whole. It consists of right and left cerebellar hemispheres connected by a narrow worm like bridge called the vermis. Each hemisphere exhibits slender, transverse, parallel folds called folia separated by shallow sulci. The cerebellum has a surface cortex of gray matter and a deeper layer of white matter. In a sagittal section, the white matter exhibits a branching, fernlike pattern called the arbor vitae. Each hemisphere has four masses of gray matter called deep nuclei embedded in the white matter. All input to the cerebellum goes to the cortex and all of its output comes from the deep nuclei.
Although the cerebellum is only about 10% of the mass of the brain, it has about 60% as much surface area as the cerebral cortex and it contains more than half of all brain neurons—about 100 billion of them. Its tiny, densely spaced granule cells are the most abundant type of neuron in the entire brain. Its most distinctive neurons, however, are the unusually large, globose Purkinje cells. These have a tremendous profusion of dendrites compressed into a single plane like a flat tree. Their axons travel to the deep nuclei, where they synapse on output neurons that issue fibers to the brainstem.
The cerebellum is connected to the brainstem by three pairs of stalks called cerebellar peduncles: a pair of inferior peduncles connected to the medulla oblongata, a pair of middle peduncles to the pons, and a pair of superior peduncles to the midbrain. These consist of thick bundles of nerve fibers that carry signals to and from the cerebellum. Connections between the cerebellum and brainstem are very complex.
Most spinal input enters the cerebellum by way of the inferior peduncles; most input from the rest of the brain enters by way of the middle peduncles; and cerebellar output travels mainly by way of the superior peduncles.
The cerebellum had come to be regarded as a center for monitoring muscle contractions and aiding in motor coordination. People with cerebellar lesions exhibit serious deficits in coordination and locomotor ability. However with fMRI and PET scan, the cerebellum’s general role in the evaluation of certain kinds of sensory input, and monitoring muscle movement is only part of its broader function. The cerebellum is highly active when a person explores objects with the fingertips, for example to compare the textures of two objects without looking at them.
Some spatial perception also resides here. The cerebellum is much more active when a person is required to solve a pegboard puzzle than when moving pegs randomly around the same puzzle board. People with cerebellar lesions also have difficulty identifying different views of a three-dimensional object as belonging to the same object. The cerebellum is also a timekeeper. An important aspect of cerebellar timekeeping is the ability to predict where a moving object will be in the next second or so.
The cerebellum also helps to predict how much the eyes must move in order to compensate for head movements and remain fixed on an object. Even hearing has some newly discovered and surprising cerebellar components. Cerebellar lesions impair a person’s ability to judge differences in pitch between two tones and to distinguish between similar-sounding words such as rabbit and rapid. Language output also involves the cerebellum. If a person is given a noun such as apple and told to think of a related verb such as eat, the cerebellum shows higher PET scan activity than if the person is told merely to repeat the word apple.
People with cerebellar lesions also have difficulty planning and scheduling tasks. They tend to overreact emotionally and have difficulty with impulse control.
Figure 12. Cerebellum of brain
The Brainstem
The brainstem is continuous with the spinal cord and consists of the medulla oblongata, pons, and midbrain.
Figure 13. The Brainstem
The Midbrain
The midbrain contains the cerebral aqueduct, continuations of the medial lemniscus and reticular formation, and the motor nuclei of two cranial nerves that control eye movements: cranial nerves III (oculomotor) and IV (trochlear).
The part of the midbrain posterior to the cerebral aqueduct is a rooflike tectum. It exhibits four bulges, the corpora quadrigemina. The upper pair, called the superior colliculi, functions in visual attention; visually tracking moving objects; such reflexes as blinking, focusing, pupillary dilation and constriction; and turning the eyes and head in response to a visual stimulus (for example, to look at something that you catch sight of in your peripheral vision). The lower pair, called the inferior colliculi, receives signals from the inner ear and relays them to other parts of the brain, especially the thalamus. Among other functions, they mediate the reflexive turning of the head in response to a sound, and one’s tendency to jump when startled by a sudden noise.
Anterior to the cerebral aqueduct, the midbrain consists mainly of the cerebral peduncles—two stalks that anchor the cerebrum to the brainstem. Each peduncle has three main components: tegmentum, substantia nigra, and cerebral crus. The tegmentum is dominated by the red nucleus, named for a pink color imparted by its high density of blood vessels. Fibers from the red nucleus its connections go mainly to and from the cerebellum, with which it collaborates in fine motor control. The substantia nigra is a dark gray to black nucleus pigmented with melanin. It is a motor center that relays inhibitory signals to the thalamus and basal nuclei (both of which are discussed later), preventing unwanted body movement. Degeneration of the neurons in the substantia nigra leads to the muscle tremors of Parkinson disease. The cerebral crus is a bundle of nerve fibers that connect the cerebrum to the pons and carry the corticospinal nerve tracts.
The cerebral aqueduct is encircled by the central (periaqueductal) gray matter. This is involved with the reticulospinal tracts in controlling awareness of pain.
Figure 14. Midbrain
The Reticular Formation
The reticular formation is a loose web of gray matter that runs vertically through all levels of the brainstem, appearing at all three levels of figure. It occupies much of the space between the white fiber tracts and the more anatomically distinct brainstem nuclei, and has connections with many areas of the cerebrum. It consists of more than 100 small neural networks defined less by anatomical boundaries than by their use of different neurotransmitters. The functions of these networks include the following:
- Somatic motor control. Some motor neurons of the cerebral cortex send their axons to reticular formation nuclei, which then give rise to the reticulospinal tracts of the spinal cord. These tracts adjust muscle tension to maintain tone, balance, and posture, especially during body movements. The reticular formation also relays signals from the eyes and ears to the cerebellum so the cerebellum can integrate visual, auditory, and vestibular (balance and motion) stimuli into its role in motor coordination. Other motor nuclei include gaze centers, which enable the eyes to track and fixate objects, and central pattern generators—neural pools that produce rhythmic signals to the muscles of breathing and swallowing.
- Cardiovascular control. The reticular formation includes the previously mentioned cardiac and vasomotor centers of the medulla oblongata.
- Pain modulation. The reticular formation is one route by which pain signals from the lower body reach the cerebral cortex. It is also the origin of the descending analgesic pathways. Under certain circumstances, the nerve fibers in these pathways act in the spinal cord to deaden one’s awareness of pain.
- Sleep and consciousness. The reticular formation has projections to the thalamus and cerebral cortex that allow it some control over what sensory signals reach the cerebrum and come to your conscious attention. It plays a central role in states of consciousness such as alertness and sleep. Injury to the reticular formation can result in irreversible coma.
- Habituation. This is a process in which the brain learns to ignore repetitive, inconsequential stimuli while remaining sensitive to others. In a noisy city, for example, a person can sleep through traffic sounds but wake promptly to the sound of an alarm clock or a crying baby. Reticular formation nuclei that modulate activity of the cerebral cortex are called the reticular activating system or extrathalamic cortical modulatory system.
The Pons
The pons measures about 2.5 cm long. Most of it appears as a broad anterior bulge rostral to the medulla. Posteriorly, it consists mainly of two pairs of thick stalks called cerebellar peduncles. They connect the cerebellum to the pons and midbrain. In cross section, the pons exhibits continuations of the previously mentioned reticular formation, medial lemniscus, tectospinal tract, and other spinal tracts. The anterior half of the pons is dominated by tracts of white matter, including transverse fascicles that cross between left and right and connect the two hemispheres of the cerebellum, and longitudinal fascicles that carry sensory and motor signals up and down the brainstem.
Cranial nerves V to VIII begin or end in the pons. The other three emerge from the groove between the pons and medulla. The functions of these four nerves, include sensory roles in hearing, equilibrium, and taste; facial sensations such as touch and pain; and motor roles in eye movement, facial expressions, chewing, swallowing, urination, and the secretion of saliva and tears. The reticular formation in the pons contains additional nuclei concerned with sleep, respiration, and
posture.
The Medulla Oblongata
The medulla oblongata extends from the pons to the foramen magnum of the skull. Its posterior surface flattens to form the floor of the fourth ventricle. Its anterior surface is marked by two longitudinal enlargements called the pyramids, which contain the corticospinal tracts. Most of the fibers of the corticospinal tracts cross over at this level. All of the ascending and descending nerve fibers connecting the brain and spinal cord must pass through the medulla oblongata because of its location. In the spinal cord the white matter surrounds a central mass of gray matter. Here in the medulla oblongata, however, nerve fibers separate the gray matter into nuclei, some of which relay ascending impulses to the other side of the brainstem and then on to higher brain centers. Other nuclei in the medulla oblongata control vital visceral activities.
These centers include:
- The cardiac center. Impulses originating in the cardiac center are conducted to the heart on peripheral nerves, altering heart rate.
- The vasomotor center. Certain neurons of the vasomotor center initiate impulses that travel to smooth muscle in the walls of certain blood vessels and stimulate the smooth muscle to contract. This constricts the blood vessels (vasoconstriction), raising blood pressure. Other neurons of the vasomotor center produce the opposite effect—dilating blood vessels (vasodilation) and consequently dropping blood pressure.
- The respiratory center. Groups of neurons in the respiratory center maintain breathing rhythm and adjust the rate and depth of breathing. Still other nuclei in the medulla oblongata are centers for the reflexes associated with coughing, sneezing, swallowing, and vomiting.
Protective Coverings of the Brain
The brain is enveloped in three membranes called the meninges, which lie between the nervous tissue and bone. They protect the brain and provide a structural framework for its arteries and veins. As in the spinal cord, these are the dura mater, arachnoid mater, and pia mater. However, the cranial dura mater consists of two layers—an outer periosteal layer equivalent to the periosteum of the cranial bones, and an inner meningeal layer. Only the meningeal layer continues into the vertebral canal, where it forms the dural sheath around the spinal cord. The cranial dura mater is pressed closely against the cranial bone, with no intervening epidural space like the one around the spinal cord. It is not attached to the bone, however, except in limited places: around the foramen magnum, the sella turcica, the crista galli, and the sutures of the skull.
Meningitis is inflammation of the meninges that surrounds the brain and spinal cord. There are several types of meningitis. The most common is viral meningitis. You get it when a virus enters the body through the nose or mouth and travels to the brain. Bacterial meningitis is rare, but can be deadly. It usually starts with bacteria that cause a cold-like infection. It can cause stroke, hearing loss, and brain damage. It can also harm other organs. Pneumococcal infections and meningococcal infections are the most common causes of bacterial meningitis. Anyone can get meningitis, but it is more common in people with weak immune systems. Meningitis can get serious very quickly.
Figure 15. Meninges of the brain
In some places, the two layers of dura are separated by dural sinuses, spaces that collect blood that has circulated through the brain. Two major, superficial ones are the superior sagittal sinus, found just under the cranium along the median line, and the transverse sinus, which runs horizontally from the rear of the head toward each ear. These sinuses meet like an inverted T at the back of the brain and ultimately empty into the internal jugular veins of the neck.
In certain places, the meningeal layer of the dura folds inward to separate major parts of the brain from each other and limit brain movements within the cranium, as when one receives a blow to the head. There are three of these: (1) the falx cerebri, which extends into the longitudinal fissure as a tough, crescent-shaped wall between the right and left cerebral hemispheres; (2) the tentorium cerebelli, which stretches like a roof over the posterior cranial fossa and separates the cerebellum from the overlying cerebrum; and (3) the falx cerebelli, a vertical partition between the right and left halves of the cerebellum on the inferior side.
The arachnoid mater and pia mater are similar to those of the spinal cord. The arachnoid mater is a transparent membrane over the brain surface, deep to the dura. A subarachnoid space separates it from the pia below, and in some places, a subdural space separates it from the dura above. The subarachnoid space contains the largest blood vessels of the cerebral surface. The pia mater is a very thin, delicate membrane, not usually visible without a microscope. Whereas the arachnoid meninx only overlies the sulci of the cerebral surface, the pia mater dips down into them and closely follows all the contours of the brain.
Ventricles and Cerebrospinal Fluid
The brain has four internal chambers called ventricles. The largest and most frontal ones are the two lateral ventricles, which form an arc in each cerebral hemisphere. Through a tiny pore called the interventricular foramen, each lateral ventricle is connected to the third ventricle, a narrow median space inferior to the corpus callosum. From here, a canal called the cerebral aqueduct passes down the core of the midbrain and leads to the fourth ventricle, a small triangular chamber between the pons and cerebellum. Caudally, this space narrows and forms a central canal that extends through the medulla oblongata into the spinal cord.
Figure 16. Ventricles of the brain
On the floor or wall of each ventricle is a spongy mass of blood capillaries called a choroid plexus, named for its histological resemblance to a fetal membrane called the chorion. Ependyma, a type of neuroglia that resembles a cuboidal epithelium, lines the ventricles and canals and covers the choroid plexuses. It produces cerebrospinal fluid (CSF).
Cerebrospinal fluid is a clear, colorless liquid that fills the ventricles and canals of the CNS and bathes its external surface. The brain produces about 500 mL of cerebrospinal fluid per day, but the fluid is constantly reabsorbed at the same rate and only 100 to 160 mL is normally present at one time. About 40% of it is formed in the subarachnoid space external to the brain, 30% by the general ependymal lining of the brain ventricles, and 30% by the choroid plexuses. Cerebrospinal fluid production begins with the filtration of blood plasma through the capillaries of the brain. Ependymal cells modify the filtrate as it passes through them, so the cerebrospinal fluid has more sodium chloride than blood plasma, but less potassium, calcium, and glucose and very little protein.
Cerebrospinal fluid serves three functions for the brain:
- Buoyancy. Because the brain and cerebrospinal fluid are similar in density, the brain neither sinks nor floats in the cerebrospinal fluid. It hangs from delicate specialized fibroblasts of the arachnoid meninx. A human brain removed from the body weighs about 1.5 kg, but when suspended in cerebrospinal fluid its effective weight is only about 50 g. This buoyancy allows the brain to attain considerable size without being impaired by its own weight. If the brain rested heavily on the floor of the cranium, the pressure would kill the nervous tissue.
- Protection. Cerebrospinal fluid also protects the brain from striking the cranium when the head is jolted. If the jolt is severe, however, the brain still may strike the inside of the cranium or suffer shearing injury from contact with the angular surfaces of the cranial floor. This is one of the common findings in head injuries (concussions) from contact sports like NFL, rugby and boxing.
- Chemical stability. Cerebrospinal fluid rinses metabolic wastes from the nervous tissue and regulates its chemical environment. Slight changes in cerebrospinal fluid composition can cause malfunctions of the nervous system. For example, a high glycine concentration disrupts the control of body temperature and blood pressure, and a high pH causes dizziness and fainting.
Cerebrospinal fluid continually flows through and around the brain and spinal cord, driven partly by its own pressure, partly by the beating of ependymal cilia, and partly by rhythmic pulsations of the brain produced by each heartbeat. The cerebrospinal fluid of the lateral ventricles flows through the interventricular foramina into the third ventricle, then down the cerebral aqueduct to the fourth ventricle. The third and fourth ventricles and their choroid plexuses add more cerebrospinal fluid along the way. A small amount of cerebrospinal fluid fills the central canal of the spinal cord, but ultimately, all of it escapes through three pores in the fourth ventricle—a median aperture and two lateral apertures. These lead into the subarachnoid space on the brain and spinal cord surface. From here, cerebrospinal fluid is reabsorbed by arachnoid granulations, extensions of the arachnoid meninx shaped like little sprigs of cauliflower, protruding through the dura mater into the superior sagittal sinus. Cerebrospinal fluid penetrates the walls of the granulations and mixes with blood in the sinus.
Figure 17. Cerebrospinal fluid formation, absorption and circulation around and within the brain
Blood Supply to the Brain and the Brain Barrier System
Although the brain is only 2% of the adult body weight, it receives 15% of the blood (about 750 mL/min.) and consumes 20% of its oxygen and glucose. Because neurons have such a high demand for ATP (adenosine triphosphate is a high-energy molecule found in every cell, its job is to store and supply the cell with needed energy) and therefore glucose and oxygen, the constancy of blood supply is especially critical to the nervous system. A mere 10-second interruption in blood flow can cause loss of consciousness; an interruption of 1 to 2 minutes can significantly impair neural function; and 4 minutes without blood usually causes
irreversible brain damage.
The brain receives its arterial supply from two pairs of vessels, the vertebral and internal carotid arteries, which are interconnected in the cranial cavity to produce a cerebral arterial circle (of Willis). The two vertebral arteries enter the cranial cavity through the foramen magnum and just inferior to the pons fuse to form the basilar artery. The two internal carotid arteries enter the cranial cavity through the carotid canals on either side.
Figure 18. Brain blood supply
Despite blood’s critical importance to the brain, blood is also a source of antibodies, macrophages, bacterial toxins, and other potentially harmful agents. Damaged brain tissue is essentially irreplaceable, and the brain therefore must be well protected. Consequently, there is a brain barrier system that strictly regulates what can get from the bloodstream into the tissue fluid of the brain.
There are two potential points of entry that must be guarded:
- Blood capillaries throughout the brain tissue and
- Capillaries of the choroid plexuses.
At the blood capillaries that are present throughout the brain tissue, the brain is well protected by the blood–brain barrier, which consists of tight junctions between the endothelial cells that form the capillary walls. In the developing brain, astrocytes reach out and contact the capillaries with their perivascular feet, stimulating the endothelial cells to form tight junctions that completely seal off the gaps between them. This ensures that anything leaving the blood must pass through the cells and not between them. The endothelial cells are more selective than gaps between them would be, and can exclude harmful substances from the brain tissue while allowing necessary ones to pass through.
At the choroid plexuses, the brain is protected by a similar blood–cerebrospinal fluid barrier formed by tight junctions between the ependymal cells. Tight junctions are absent from ependymal cells elsewhere, because it is important to allow exchanges between the brain tissue and cerebrospinal fluid. That is, there is no brain–cerebrospinal fluid barrier.
The blood–brain barrier is highly permeable to water, glucose, and lipid soluble substances such as oxygen, carbon dioxide, alcohol, caffeine, nicotine, and anesthetics. It is slightly permeable to sodium, potassium, chloride, and the waste products urea and creatinine. While the blood–brain barrier is an important protective device, it is an obstacle to the delivery of medications such as antibiotics and cancer drugs, and thus complicates the treatment of brain diseases.
Trauma and inflammation sometimes damage the blood–brain barrier and allow pathogens to enter the brain tissue. Furthermore, there are places called circumventricular organs in the third and fourth ventricles where the barrier is absent and the blood has direct access to brain neurons. These enable the brain to monitor and respond to fluctuations in blood glucose, pH, osmolarity, and other variables. Unfortunately, circumventricular organs also afford a route of invasion by pathogens.
References- National Center for Biotechnology Information, U.S. National Library of Medicine. Hypothalamus. https://www.ncbi.nlm.nih.gov/pubmedhealth/PMHT0022790/
- Lemaire J-J, Nezzar H, Sakka L, et al. Maps of the adult human hypothalamus. Surgical Neurology International. 2013;4(Suppl 3):S156-S163. doi:10.4103/2152-7806.110667. https://www.ncbi.nlm.nih.gov/pmc/articles/PMC3654779/