What is glucagon
Glucagon is a hormone produced in the pancreas alpha cells in the islets of Langerhans and glucagon is secreted between meals when your blood glucose concentration falls below 100 mg/dL (5.6 mmol/L). The main physiological role of glucagon is to stimulate liver glucose output, thereby leading to increases in glycemia. This provides the major counterregulatory mechanism for insulin in maintaining glucose homeostasis 1. Glucagon exerts two primary actions on your liver: (1) glycogenolysis, the breakdown of glycogen into glucose; and (2) gluconeogenesis, the synthesis of glucose from fats and proteins. These effects lead to the release of glucose into circulation, thus raising the blood glucose level. In adipose tissue, glucagon stimulates fat catabolism (breakdown) and the release of free fatty acids. Glucagon is also secreted in response to rising amino acid levels in the blood after a high-protein meal. Glucagon promotes amino acid absorption and thereby provides cells with the raw material for gluconeogenesis. The secretion of glucagon is inhibited by hyperglycemia, insulin, somatostatin, and glucagon like-peptide-1 (GLP-1) 2.
Glucagon is also used in diagnostic testing of the stomach and other digestive organs. Glucagon function is to defend against decreases in glucose availability during fasting, stress, and exercise by stimulating liver glycogenolysis (glycogen breakdown) phasically and liver gluconeogenesis (glucose production) tonically 2.
Insulin vs glucagon
Insulin, “the hormone of nutrient abundance,” is secreted during and immediately following a meal when blood nutrient levels are rising. Insulin is a hormone that helps glucose get into the body’s cells where it can be used for energy. Without insulin around, glucose stays in the blood and blood sugar levels get too high. Insulin plays an important role to keep plasma glucose value within a relatively narrow range throughout the day (glucose homeostasis). Insulin’s main actions are (1) In the liver, insulin promotes glycolysis and storage of glucose as glycogen (glycogenesis), as well as conversion of glucose to triglycerides, (2) In muscle, insulin promotes the uptake of glucose and its storage as glycogen, and (3) in adipose tissue, insulin promotes uptake of glucose and its conversion to triglycerides for storage. Insulin is secreted by Beta (β) cells, or B cells of islets of the Langerhans in your pancreas.
The effects of glucagon are the opposite of the effects induced by insulin. The two hormones need to work in partnership with each other to keep blood glucose levels balanced.
In times of plenty, insulin stimulates cells to absorb glucose, fatty acids, and amino acids and to store or metabolize them; therefore, it lowers the level of blood glucose and other nutrients. It promotes the synthesis of glycogen, fat, and protein, thereby promoting the storage of excess nutrients for later use and enhancing cellular growth and differentiation. It also antagonizes glucagon, thus suppressing the use of already-stored fuels. The brain, liver, kidneys, and red blood cells absorb and use glucose without need of insulin, but insulin does promote glycogen synthesis in the liver. Insulin insufficiency or inaction is well known as the cause of diabetes. Osteocalcin, a hormone from the osteoblasts of bone, also stimulates multiplication of beta cells, insulin secretion, and insulin sensitivity of other body tissues. The principal targets of insulin are the liver, skeletal muscles, and adipose tissue.
Glucagon vs glycogen
Glycogen is a stored form of glucose. Glycogen is a large multi-branched polymer of glucose which is accumulated in response to insulin and broken down into glucose in response to glucagon.
Glycogen is mainly stored in the liver and the muscles and provides the body with a readily available source of energy if blood glucose levels decrease.
The role of glycogen
Energy can be stored by the body in different forms. One form of stored energy is fat and glycogen is another. Fatty acids are more energy rich but glucose is the preferred energy source for your brain and glucose also can provide energy for cells in the absence of oxygen, for instance during anaerobic exercise.
Glycogen is therefore useful for providing a readily available source of glucose for the body.
Glycogen storage in diabetes
In a healthy body, the pancreas will respond to higher levels of blood glucose, such as in response to eating, by releasing insulin which will lower blood glucose levels by prompting the liver and muscles to take up glucose from the blood and store it as glycogen.
People with diabetes either do not make enough of their own insulin and/or their insulin does not work effectively enough. As a result, the pancreas may not be able to respond effectively enough to rises in blood glucose.
Glycogen release
Glycogen may be released by the liver for a number of reasons, including:
- In response to stressful situations
- Upon waking (this process is known as the dawn phenomenon)
- In response to low blood sugar
- To aid digestion
In these situations, when the body feels extra glucose is needed in the blood, the pancreas will release the hormone glucagon which triggers the conversion of glycogen into glucose for release into the bloodstream.
Glycogen and exercise
Glycogen plays an important role in keeping your muscles fueled for exercise. When you exercise, your muscles will take advantage of their stored glycogen. Glucose in your blood and glycogen stored in the liver can also be used to keep your muscles fueled.
Once you complete your exercise session, your muscles will replenish their glycogen stores. The time it takes to fully replenish glycogen stores can depend on how hard and how long you exercise and can vary from a few hours to several days.
Where is glucagon produced
The main site of glucagon production is pancreatic Alpha (α) cells, or A cells of islets of the Langerhans in your pancreas, which secrete glucagon in response to hypoglycemia (low blood sugar), amino acids, gastric inhibitory peptide, and ghrelin. In humans, A-cells are scattered throughout the islets of Langerhans. The pancreatic content of glucagon varies considerably among species; human pancreas contains approximately 700 to 1000 µg of glucagon, roughly 1-2 x 1017 molecules 3. For reference, the normal human pancreas contains about ~150 U of insulin or ~5000 µg 4; this translates to ~6-8 x 1017 molecules. Thus the normal human pancreas has about 1/3 to 1/10 the number of glucagon molecules as insulin molecules 3.
Figure 1. Pancreas location
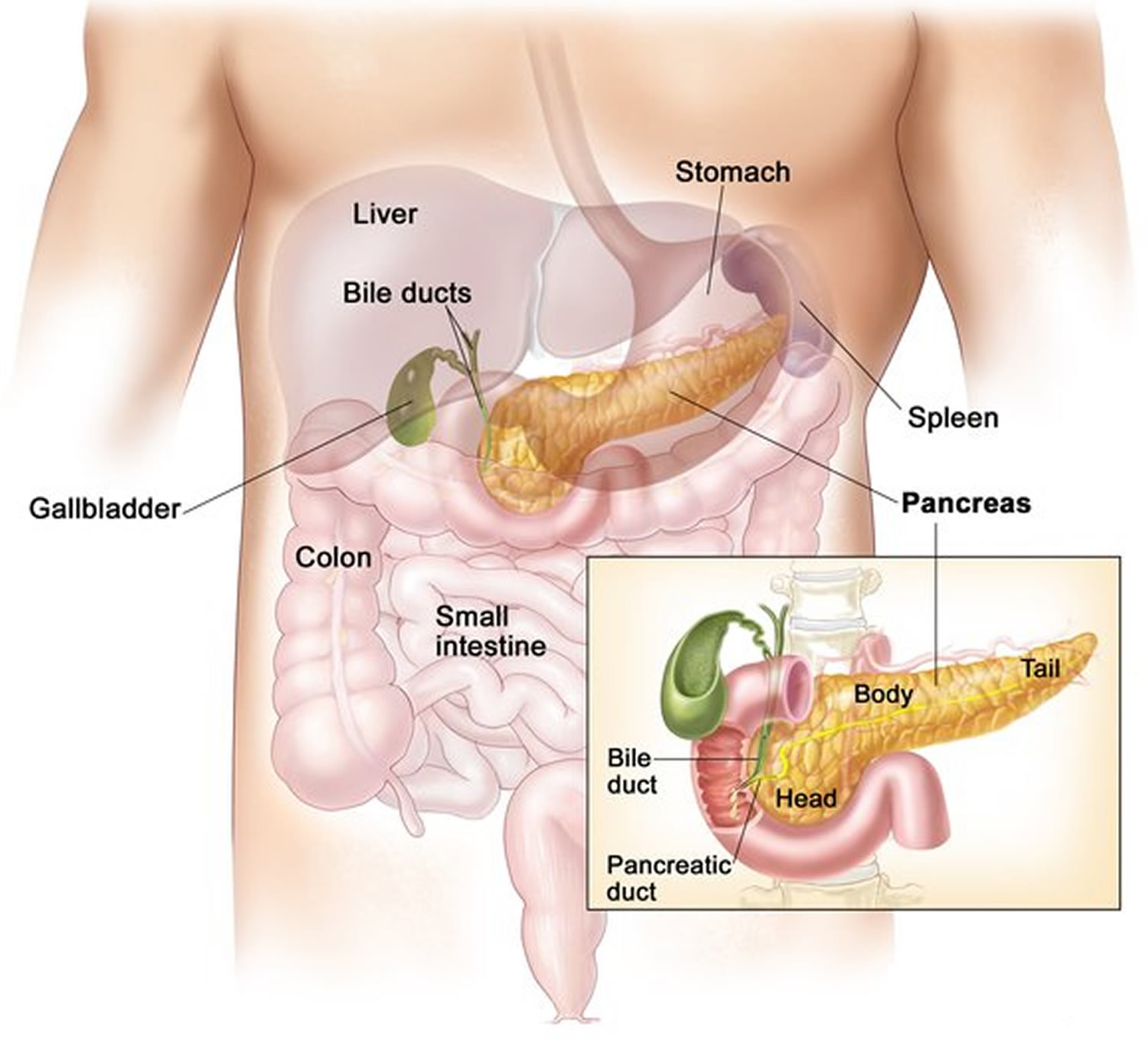
What does glucagon do
Glucagon is a single-chain polypeptide made up of 29 amino acids that are derived from a larger precursor peptide, which is cleaved upon secretion. Glucagon acts exclusively on the liver to antagonize insulin effects on hepatocytes (liver cells). Glucagon generally elevates the concentration of glucose in your blood by promoting gluconeogenesis and glycogenolysis by stimulating the cAMP pathway. Glucagon also promotes oxidation of fat (lipolysis), which can lead to the formation of ketone bodies. Glucagon also causes relaxation of the smooth muscle of the stomach, duodenum, small bowel, and colon. The effects of glucagon are the opposite of the effects induced by insulin. The two hormones need to work in partnership with each other to keep blood glucose levels balanced.
At concentrations that approximate those found in the portal vein in vivo, glucagon is a potent stimulator of hepatic glycogenolysis, gluconeogenesis, and ketogenesis in vitro. These actions of glucagon and the increases in plasma glucagon observed during hypoglycemia 5, exercise 6, trauma 7, infection (72), and other stress 8 provide considerable evidence that glucagon is important in the maintenance of euglycemia in the postabsorptive state and at times when there are increased demands for fuels and when the organism must rely on mobilization of endogenous substrate. Under these conditions, when B-cell function is normal, the major action of glucagon would be to counteract the actions of insulin on storage of glucose and other fuels. Conversely, when B-cell function is deficient, glucagon could accentuate the metabolic consequences of insulin deficiency and be an important determinant of the magnitude of hyperglycemia and hyperketonemia found in diabetes 3.
In addition to a role for glucagon in the maintenance of normal blood glucose by antagonizing the effects of postabsorptive (i.e. low) plasma insulin concentrations, there is considerable evidence that glucagon acts in the defense against hypoglycemia by antagonizing the effects of excess plasma insulin 9. When hypoglycemia is produced in humans by injection of insulin, release of glucagon is stimulated along with that of other counterregulatory hormones when the plasma glucose decreases below 3.8 mmol/L (~68 mg/dl). Restoration of normal blood glucose is due to a compensatory increase in hepatic glucose production. Although secretion of catecholamines, growth hormone, and cortisol are stimulated along with that of glucagon, only the increases in plasma glucagon and catecholamines coincide with or precede the compensatory increase in the glucose production rate 10. That glucagon is the major acute glucose counterregulatory hormone is suggested by the fact that inhibition of the plasma glucagon responses by somatostatin markedly attenuates the compensatory increase in the glucose production rate and impairs restoration of euglycemia following insulin administration. Prevention of cortisol secretion 11, adrenergic blockade 10, adrenalectomy 9, or acute growth hormone deficiency 10 does not appreciably affect immediate glucose counterregulation. The effects of glucagon during restoration of euglycemia involves both glycogenolysis and gluconeogenesis, predominantly the former 12.
Glucagon acts via the glucagon receptor, a seven transmembrane G protein-coupled receptor, located in the plasma membrane consisting of 485 amino acids 13. To date, glucagon-binding sites have been identified in multiple tissues, including liver, brain, pancreas, kidney, intestine, and adipose tissues 14. The conformation change in the receptor activates G proteins, a heterotrimeric protein with α, β, and γ subunits. When the G protein interacts with the receptor, it undergoes a conformational change that results in the replacement of the GDP molecule that was bound to the α subunit with a GTP molecule. This substitution results in the releasing of the α subunit from the β and γ subunits. The alpha subunit specifically activates the next enzyme in the cascade, adenylate cyclase.
Adenylate cyclase manufactures cyclic adenosine monophosphate (cyclic AMP or cAMP), which activates protein kinase A (cAMP-dependent protein kinase). This enzyme, in turn, activates phosphorylase kinase, which then phosphorylates glycogen phosphorylase b (PYG b), converting it into the active form called phosphorylase a (PYG a). Phosphorylase a is the enzyme responsible for the release of glucose-1-phosphate from glycogen polymers.
Additionally, the coordinated control of glycolysis and gluconeogenesis in the liver is adjusted by the phosphorylation state of the enzymes that catalyze the formation of a potent activator of glycolysis called fructose-2,6-bisphosphate 15. The enzyme protein kinase A (PKA) that was stimulated by the cascade initiated by glucagon will also phosphorylate a single serine residue of the bifunctional polypeptide chain containing both the enzymes fructose-2,6-bisphosphatase and phosphofructokinase-2. This covalent phosphorylation initiated by glucagon activates the former and inhibits the latter. This regulates the reaction catalyzing fructose-2,6-bisphosphate (a potent activator of phosphofructokinase-1, the enzyme that is the primary regulatory step of glycolysis) 16 by slowing the rate of its formation, thereby inhibiting the flux of the glycolysis pathway and allowing gluconeogenesis to predominate. This process is reversible in the absence of glucagon (and thus, the presence of insulin).
Glucagon stimulation of protein kinase A also inactivates the glycolytic enzyme pyruvate kinase in hepatocytes 17.
Glucagon regulation
Glucagon secretion, similar to that of insulin, is tightly regulated and intimately tied to blood glucose levels (Figure 3) 3. Converse to the inhibition of insulin secretion by hypoglycemia, low levels of blood glucose directly stimulate the pancreatic alpha (α) cells to secrete glucagon 18. Hyperglycemia decreases glucagon secretion 5. In vitro studies, such as those using the isolated perfused pancreas in which most variables operative in vivo can be controlled, indicate that the alpha (α) cells is as exquisitely sensitive to changes in the ambient extracellular glucose concentration as is the beta-cell 5; thus, glucose suppresses basal and stimulated glucagon release at concentrations as low as 5 mmol/L glucose (90 mg/dl). To some extent the inhibition of glucagon secretion is dependent on concomitant stimulation of insulin release. In vivo, a decrease in plasma glucose of 1 to 2 mmol/L increases plasma glucagon 19.
The secretion of glucagon is promoted via the action of voltage-dependent sodium (Na+) and calcium (Ca 2+) channels, which maintain action potentials during times of low glucose. Depolarization increases the Ca 2+ influx and subsequent glucagon secretion 20, which is supported by the activity of ATP-sensitive potassium (KATP) channels 21. As glucose levels rise, secretion of glucagon is inhibited through the elevation of cytosolic ATP, blockade of KATP channels and termination of the Na+-induced and Ca2+-induced action potentials. This process inhibits Ca2+ influx and ends glucagon secretion. Although the cellular signals regulating glucagon secretion are fairly well-established, the role of glucose, whether directly, or indirectly via β-cell activation, is still a matter of debate. Studies in rats suggest that mediatory, paracrine signaling from the β cell is essential for the inhibition of glucagon secretion by elevated glucose levels 22. Investigations in mice and humans, however, suggest that glucose directly inhibits glucagon secretion at concentrations which are too low to stimulate insulin secretion 21. Furthermore, this inhibition has been demonstrated in vitro in both isolated α cells and in intact pancreatic islets 23. However, a study in individuals with type 1 diabetes mellitus suggests that insulin is the primary signal that inhibits glucagon secretion in humans 24. In addition to glucose, several other physiological parameters are known regulators of glucagon secretion, including GLP-1 25, GLP-2 26, fatty acids 27, the autonomic nervous system 28 and circulating amino acids 29.
Figure 3. Some controls of glucagon secretion
Footnote: Nutrient, neural, endocrine, paracrine, and autocrine effects control glucagon secretion. The dynamic interactions among these controls are poorly understood. As the figure indicates, there are many possibilities for positive- and negative-feedback loops (e.g., glucagon stimulates both hepatic glucose production and insulin secretion, and both glucose and insulin inhibit glucagon secretion), for indirect effects (e.g., the incretin hormone glucagon-like peptide 1 [GLP-1] stimulates insulin and amylin secretion, both of which inhibit glucagon secretion, both by acting directly on the α-cells and by reducing gastric emptying), and for antagonistic effects (one example is that the incretin hormone GLP-1 inhibits glucagon secretion but the incretin hormone glucose-dependent insulinotropic polypeptide [GIP] stimulates it; another example is that mixed-nutrient meals increase both plasma glucose, which inhibits glucagon secretion, and plasma amino acids, which stimulates glucagon secretion, with the net result a brief stimulation of glucagon secretion). Somatostatin may contribute in situations in which insulin and glucagon secretion do not change in a simple inverse fashion, such as after mixed-nutrient meals. Finally, central and peripheral glucose-sensing neurons converge onto sympathetic and vagal efferents that drive insulin and glucagon secretion. α, pancreatic α-cell (glucagon); β, pancreatic β-cell (insulin); δ, pancreatic δ-cell (somatostatin); arrows, stimulatory inputs; T-ends, inhibitory inputs.
[Source 30 ]Glucagon and lipid metabolism
Plasma lipid homeostasis
Studies in the early 1960s investigated glucagon’s action independent of glucose homeostasis. These studies described a lipid-mobilizing effect for glucagon in a range of species 31. The regulation of plasma lipids by glucagon was first highlighted in studies which suggested that glucagon acts to decrease plasma cholesterol, total esterified fatty acids and arachidonic acid levels 32. As epinephrine, insulin and exogenous glucose all failed to lower levels of plasma cholesterol, the investigators concluded that these effects were not an indirect result of altered carbohydrate metabolism 32. In both canines and humans, the researchers observed a total decrease in levels of plasma cholesterol, as well as plasma total lipid concentrations, within 30 min of intravenous glucagon administration. Intriguingly, this depression was not found for whole-blood total lipid levels, suggesting a repartitioning of lipids from serum to platelet-rich fractions. Incubation of the blood at 37 °C led to partial or full restoration of plasma cholesterol and total lipid levels, further supporting the hypothesis of repartitioning 33. Reports from other groups provided additional support for a role of glucagon in the regulation of plasma lipids 34.
In the context of triglyceride metabolism, several reports indicated that triglyceride production is decreased in perfused livers treated with glucagon 35. Although these studies suggest hepatic lipoprotein metabolism as the site of such regulation, the molecular mechanism by which glucagon achieved this result remained elusive. Building upon these observations, the role of glucagon on lipid suppression was investigated in a rat model of hyperlipidemia 36. This study demonstrated that glucagon sig nificantly decreased levels of serum triglycerides, cholesterol, and VLDL cholesterol in hyperlipidemic rats, as well as in control eulipidemic rats. Furthermore, a decrease in synthesis of hepatic lipoprotein apoproteins was observed 36, complementing earlier studies that described depression of triglyceride synthesis in livers treated with glucagon. A caveat of this study is that it involved supraphysiological concentrations of glucagon administered over a 4-day period. Thus, the relevance of acute signaling by endogenous glucagon remained uncertain. Guettet et al. 37 continued the investigation of chronic effects of glucagon signaling in the Wistar rat. Their initial studies described a decrease in concentrations of plasma cholesterol, phospholipids and tri glycerides. Interestingly, these reductions were not seen in the liver or in erythrocytes of these rats, which suggested another target tissue as the site of action. Continued investigation of cholesterol turnover revealed increased urinary secretion of cholesterol, as well as elevated transformation to bile acids. Of special importance was the reported observation that the chronic treatment of Wistar rats with twice-daily glucagon (20 μg per day) for 3 weeks reduced blood glucose and insulin concentrations. This report thus indicated that chronic glucagon pharmacology was neither transient nor detrimental to glucose homeostasis 37.
Further studies by Guettet et al. 38 evaluated the effect of chronic glucagon treatment on lipoprotein composition in fed and fasted rats, as well as rats fed a cholesterol-rich diet. Cholesterol, phospholipids and total protein levels were proportionally decreased in chylomicrons, VLDL cholesterol, LDL cholesterol and HDL cholesterol, which suggested a reduction in the number of lipoprotein particles. Triglycerides were reported to be decreased only in the chylomicron and VLDL fractions. Evaluation of the lipoproteins indicated a decline in levels of apolipoprotein E (ApoE) and an increase in ApoB 39. Collectively, these findings suggested glucagon-stimulated targeting of the ApoE-rich lipoproteins to limit the accumulation that typically occurs with high-cholesterol feeding 38. A follow-up study showed that glucagon treatment had no effect on secretion rates of triglyceride-rich particles in either fed or fasted conditions. Conversely, glucagon treatment accelerated the rate of triglyceride removal (fractional catabolic rate constant) from the plasma compartment 40. Taken together, the work of Guettet et al. 38 revealed that increased glucagon signaling may directly regulate lipid catabolism.
Rudling and Angelin 41 extended these findings by the direct study of glucagon’s effects on LDL receptors (LDLRs). The investigators found that glucagon administra tion to rats resulted in a dose-dependent increase in binding of LDL cholesterol to the receptor, with no apparent effect on receptor mRNA expression. Concomitant with this increased binding was a decrease in levels of cholesterol, ApoB, and ApoE. Furthermore, these effects were completely antagonized by insulin administration. Rudling and Angelin hypothesized a mechanism such as post-translational receptor modification, whereby glucagon increases LDLR activity without affecting expression levels 41. The findings in rodents were complemented by studies in dairy cattle which showed that subcutaneous injections of glucagon led to variable decreases, some of sizable magnitude, in circulating plasma concentrations of VLDL-triglycerides, HDL1-phospholipids and HDL2-free cholesterol 42. Improved glucose status with decreased nonesterified fatty acids and β-hydoxybutyrate was also reported 43.
Genetic manipulation of the glucagon receptor has led to inconclusive results regarding glucagon’s role in lipid metabolism. Conarello et al. 44 found that Gcgr−/− mice are resistant to high fat diet-induced liver steatosis; however, Longuet et al. 45 showed that Gcgr−/− mice have enhanced susceptibility to hepatic steatosis. Interestingly, these studies used genetically modified null mice generated in an identical manner and of the same background (C57B6). Furthermore, the effect was observed in mice fed a similar diet (45% fat). The length of diet differed between the two studies (8 versus 12 weeks); however, mice fed a high fat diet for the longer duration did not exhibit steatosis. Taken together, the contribution of glucagon receptor agonism to liver steatosis has yet to be conclusively described.
Longuet et al. 45 also demonstrated the importance of glucagon signaling in the adaptive response to fasting. Following exposure to a prolonged fasting period, Gcgr−/− mice showed increased levels of plasma triglycerides and free fatty acids 45. During either glucagon treatment or a prolonged fast, wild-type mice had an increased expression of genes involved in fatty acid oxidation, including Decr2, carnitine O-palmitoyltransferase (Cpt) 1a, Cpt2 and Acadm. These changes in expression were correlated with an increased capacity of the liver to oxidize free fatty acids. By contrast, this increased gene expression was not observed in Gcgr−/− mice, which displayed a reduced oxidation of free fatty acids in both the fed and the fasted state. These data highlight the essential physiological role of glucagon-receptor signaling in the regulation of gene expression during prolonged fasting.
Glucagon-mediated lipolysis and ketogenesis
Glucagon-mediated regulation of lipid metabolism is not limited to plasma triglycerides and cholesterol. Glucagon treatments as low as 10−8 mol/l have been implicated in promoting lipolysis in white adipose tissue 46. This lipolytic effect appears to be independent of sympathetic nervous system innervation 47, as denervation of white adipose tissue does not block glucagon-induced glycerol release, whereas it decreases the release of nonesterified fatty acids. The latter, however, is reported to be the result of re-esterification 47, not a blockade of glucagon’s lipolytic effects. Mechanistically, glucagon is known to stimulate the activity of hormone-sensitive lipase in adipocytes, resulting in an increase of nonesterified fatty acids in the circulation 48. These fatty acids, usually bound to albumin, are transported to the heart, skeletal muscle, kidney and liver, where they are catabolyzed or, in the case of the liver, alternatively converted to ketone bodies.
Ketone bodies provide up to two-thirds of the energy for the brain during times of glucose deficiency, thus sparing glucose utilization and reducing proteolysis 49. Supporting a role for glucagon in the regulation of keto-genesis, suppression of glucagon secretion via somatostatin prevented the development of ketoacidosis in patients with type 1 diabetes mellitus 50. Pegorier et al. 51 elucidated the regulation of fatty acid oxidation and keto-genesis by glucagon in rabbit hepatocytes. The researchers reported increased ketone-body production in the presence of either glucagon or dibutyryl cAMP. Oxidation of exogenous oleate was increased by both glucagon and cAMP, effects which were reduced in the presence of insulin. Furthermore, exposure to glucagon or cAMP completely inhibited lipogenesis and decreased malonyl-CoA concentration and stimulated fatty acid oxidation. These effects were suggested to be driven by a fall in the sensitivity of CPT1 to malonyl-CoA, releasing the inhibition of this rate-limiting enzyme in the transport of fatty acids across the mitochondrial membranes 51. The relevance of these studies was confirmed by the observation that glucagon directly controls ketone-body production in primary human hepatocytes 52. Specifically, glucagon increased ketone-body production and fatty acid oxidation, whereas it decreased fatty acid esterification, similar to the effects of cAMP 52.
Lipid-induced glucagon resistance
In addition to glucagon’s regulation of lipid metabolism, previous studies suggest that lipids may also regulate glucagon signaling. Studies by Charbonneau et al. 53 show that rats fed a high fat diet display hepatic steatosis that is associated with glucagon resistance. Specifically, exercise training in rats was used to increase plasma glucagon levels and improve fatty liver. In trained rats, a negative correlation between liver adiposity and density of the glucagon receptors in the plasma membrane was observed. Additional studies described a reduction in both hepatic glucagon-receptor density and Gsα protein content at the plasma membrane 54. The mechanism for this decrease in receptor density was explored in a study which proposed that high fat diet promoted glucagon receptor proteolysis 54. A reduction in glucagon receptors at the plasma membrane was notable, accompanied by a marked increase in the number of endosomal and lysosomal compartments. These effects were correlated with an increase of protein kinase Cα (PKCα) on the plasma membrane, which is known to phosphorylate G-protein-related kinases. Such action, in turn, is known to inhibit receptor internalization which leads to receptor desensitiza tion 55. The same association seems to be conserved in humans, as glucagon resistance is associated with pathological hyperlipidemia in humans 56.
Glucagon and bile acid metabolism
Although the canonical role of bile acids is associated with the absorption of dietary lipids and cholesterol homeostasis, new studies suggest that these acids have additional signaling roles in glucose homeostasis. This emerging function was highlighted in a study by Song and Chiang 57, who investigated cholesterol 7-alpha-monooxygenase (CYP7A1) as a possible target of glucagon signaling. Their study demonstrated that glucagon, as well as cAMP, represses CYP7A1 expression in human primary hepatocytes. CYP7A1 is the rate-limiting enzyme in bile acid synthesis, and its expression is tightly regulated as a means to control flux through the pathway 58. Song and Chiang86 further showed that glucagon’s regulation of CYP7A1 expression is mediated via PKA signaling to HNF-4α. Given that no direct measure of bile acid synthesis or secretion was available, the direct result on bile acid metabolism remains unknown. The findings of their earlier study 57, however, were complemented by work from Hylemon et al., 59 who showed similar decreases in CYP7A1 expression in response to glucagon or cAMP exposure in rat hepatocytes. These in vitro studies support initial observations that suggest a role for glucagon in the regulation of bile acid metabolism and provide a plausible mechanism for the glucagon-induced decrease in plasma cholesterol levels.
Glucagon and energy expenditure
In addition to regulating glucose and lipid metabolism, glucagon participates in the control of energy expenditure and thermogenesis. Early studies by Davidson et al. 60 showed that pharmacological infusion of glucagon increased oxygen consumption in rats. This effect was mirrored by a report in human study participants, in whom infusion of a pharmacological dose of glucagon increased resting energy expenditure during acute insulin deficiency produced by the additional infusion of somatostatin 61. Moreover, a physiological dose of glucagon increased energy expenditure in humans during euinsulinemia, and hyperinsulinemia blunted glucagon’s thermogenic activity 62. Studies conducted in vitro showed that hyperglucagonemia may increase energy expenditure via stimulation of oxygen consumption and heat production in brown adipose tissue 63. Studies in rats confirmed that glucagon administration increased whole-body oxygen consumption, core body temperature, blood flow 64, as well as temperature and mass of brown adipose tissue 65. Furthermore, cold exposure was found to increase plasma glucagon levels 66, which implicates glucagon in nonshivering thermogenesis. The stimulation of thermogenic activity in brown adipose tissue by glucagon 67 has become even more relevant, as novel findings suggest a renewed importance for brown adipose tissue in human energy metabolism 68.
The mechanism by which glucagon-induced thermogenesis is regulated is probably complex and may involve the sympathetic nervous system. Supporting this hypothesis, glucagon-induced increases in oxygen consumption, blood flow and thermogenesis in brown adipose tissue were blocked by the nonselective β adrenergic receptor blocker, propranolol 69, whereas denervation of brown adipose tissue blunted the thermogenic action of glucagon 67. Furthermore, although glucagon injection in ducklings was associated with an increase in norepinephrine, blockade of catecholamines via guanethidine sympathectomy (the interruption of the transmission of sympathetic nerve impulses by a chemical agent that blocks the secretion of epinephrine and norepinephrine at postganglionic nerve endings) decreased metabolic rate compared to nonsympathectomized birds treated with glucagon or saline 70. Glucagon might also have a direct effect on thermogenesis, as glucagon incubation of brown adipocytes of rat and mice, but not those of Syrian hamsters, markedly increased oxygen consumption 69. However, the concentration of glucagon required for such in vitro effects has been speculated to be supraphysiological 69.
The role of glucagon in mediating cold-induced thermogenic responses has been further questioned by a report that denervated interscapular brown adipose tissue decreased the number of glucagon receptors as compared to the contralateral adipose tissue pad that served as an internal (within animal) control 71. Given that cold exposure is a well-known stimulator of the sympathetic nervous system in brown adipose tissue 72, a decrease in glucagon receptor protein or gene expression mitigates the role for glucagon in cold-triggered brown adipose tissue thermogenesis via innervation of the sympathetic nervous system. Taken together, these data suggest a pivotal role for the sympathetic nervous system in glucagon-induced thermogenesis and suggest a thermogenic basis for anti-obesity effects of glucagon.
Glucagon on body weight
Recent studies have suggested that glucagon may have role in body weight regulation 3. Mice with the whole body glucagon receptor knockout have higher lean body mass despite similar to wild type animal growth pattern, food intake and energy expenditure 73. How the loss of glucagon action affects body composition remains to be determined but possible explanations may include effects on leptin, sympathetic nervous system, and brown adipose tissue thermogenesis and white adipose tissue lipolysis 74.
A study by Geary, N. & Smith, G. P. 75, found that selective hepatic vagotomy blocks glucagon’s satiety effect. This suggests a critical action of glucagon being conducted via the hepatic branch of the abdominal vagus. Although current evidence indicates that the inhibitory effect of glucagon on feeding probably arises, at least in part, from a hepatic metabolic action of the hormone, novel studies in animals suggest that additional effects of glucagon acting directly in the central nervous system to inhibit food intake are also possible 76.
Glucagon and regulation of food intake
Early studies indicated that glucagon administration diminishes the sense of hunger and decreases food intake in humans 77 and rats 78. Additional studies in rats confirmed that glucagon specifically decreases meal size owing to increased satiation rather than illness or aversion. Intravenous infusions similarly produced a dose-related decrease in eating 79. Evidence for an endogenous role of glucagon in satiation was provided by demonstrations that glucagon concentration increases physiologically during meals, in many situations 80, and that antagonism of glucagon by preprandial administration of glucagon antibodies increases the amount of food consumed in one meal (meal size) 81. Finally, intravenous infusion of a physiological dose of glucagon during meals was shown to reduce meal size in humans 82.
A study in rats with both hepatic portal vein and vena cava infusion catheters indicated that both exogenous and endogenous glucagon act in the liver to limit meal size 83. Furthermore, as for several other peripheral signals that control eating, vagal afferents relay the signal to the brain 84. In the case of glucagon, the hepatic branch of the abdominal vagus is critical 85, consistent with the hepatic site of action. Although the inhibitory effect of glucagon on feeding probably arises, at least in part, from a hepatic metabolic action of the hormone, novel studies in sheep suggest that glucagon acts directly in the central nervous system to inhibit food intake 86.
Genetically modified animal models also demonstrate that glucagon signaling is involved in regulating food intake and body composition. Gcgr−/− mice display a decreased adipose tissue mass and an increased lean mass despite similar food intake and body weight compared to their wild-type littermates 87. Furthermore, Gcgr−/− mice on a high fat diet are resistant to diet-induced obesity 44, which can be primarily attributed to a lower food intake compared with controls.
Glucagon injection
Glucagon injection is an emergency medicine used to treat severe hypoglycemia (low blood sugar) in diabetes patients treated with insulin who have passed out or cannot take some form of sugar by mouth or or treatment by mouth has not been successful. Glucagon emergency kit is available only with your doctor’s prescription. Glucagon is a hormone which helps to raise blood glucose levels. When injected, glucagon is absorbed into the blood stream and travels to the liver where it signals the liver to release glucose into the blood. Glucagon can be expected to take about 10-15 minutes to raise blood glucose back to safer levels.
Always read the instructions of the kit carefully when using glucagon.
Glucagon injection is also used as a diagnostic aid during X-ray tests of the stomach and bowels. This is to improve test results by relaxing the muscles of the stomach and bowels.
Glucagon emergency kit is available only with your doctor’s prescription. is available in the following dosage forms:
- Powder for Solution
The glucagon emergency kit will usually consist of a syringe, a vial of powder and a vial of liquid. The instructions in the kit will give details of how to mix these for injection.
- Glucagon can be injected into the arm, thigh or buttocks.
- There is no danger of overdose with glucagon.
- Glucagon can cause vomiting so make sure the patient remains in the recovery position to prevent the chances of choking.
The main symptoms associated with hypoglycemia are:
- Sweating
- Fatigue
- Feeling dizzy
Symptoms of hypoglycemia can also include:
- Being pale
- Feeling weak
- Feeling hungry
- A higher heart rate than usual
- Blurred vision
- Confusion
- Convulsions
- Loss of consciousness
- And in extreme cases, coma
Whilst medication is the main factor involved in hypoglycemia within people with diabetes, a number of other factors can increase the risk of hypos occurring.
Factors linked to a greater risk of hypos include:
- Too high a dose of medication (insulin or hypo causing tablets)
- Delayed meals
- Exercise
- Alcohol
Figure 4. Glucagon emergency kit
Glucagon is usually given by injection beneath the skin, in the muscle, or in the vein. It comes as a powder and liquid that will need to be mixed just before administering the dose. Instructions for mixing and giving the injection are in the package. Glucagon should be administered as soon as possible after discovering that the patient is unconscious from low blood sugar. After the injection, the patient should be turned onto the side to prevent choking if they vomit. Once the glucagon has been given, contact your doctor. It is very important that all patients have a household member who knows the symptoms of low blood sugar and how to administer glucagon.
If you have low blood sugar often, keep a glucagon kit with you at all times. You should be able to recognize some of the signs and symptoms of low blood sugar (i.e., shakiness, dizziness or lightheadedness, sweating, confusion, nervousness or irritability, sudden changes in behavior or mood, headache, numbness or tingling around the mouth, weakness, pale skin, sudden hunger, clumsy or jerky movements). Try to eat or drink a food or beverage with sugar in it, such as hard candy or fruit juice, before it is necessary to administer glucagon.
Follow the directions on your prescription label carefully, and ask your pharmacist or doctor to explain any part you or your household members do not understand. Use glucagon exactly as directed. Do not use more or less of it or use it more often than prescribed by your doctor.
Glucagon injection is an emergency medicine and must be used only as directed by your doctor. Make sure that you and a member of your family or a friend understand exactly when and how to use this medicine before it is needed.
This medicine comes with patient instructions together with the kit provided with the package. Read and follow the instructions carefully and ask your doctor if you have any questions.
Do not use this medicine if a gel has formed, or if you see particles in the mixed solution.
Call for emergency medical help right after receiving glucagon injection.
Drink a fast-acting source of sugar such as a regular soft drink or fruit juice, and eat a long-acting source of sugar (such as crackers and cheese or a meat sandwich) as soon as you are able to swallow.
Special precautions
Before using glucagon:
- tell your doctor and pharmacist if you are allergic to glucagon, any other drugs, or beef or pork products.
- tell your doctor and pharmacist what prescription and nonprescription medications you are taking, including vitamins.
- tell your doctor if you have ever had adrenal gland problems, blood vessel disease, malnutrition, pancreatic tumors, insulinoma, or pheochromocytoma.
- tell your doctor if you are pregnant, plan to become pregnant, or are breast-feeding.
Patients with diabetes should be aware of the symptoms of hypoglycemia (low blood sugar). These symptoms may develop in a very short time and may result from:
- using too much insulin (“insulin reaction”) or as a side effect from oral antidiabetic medicines
- delaying or missing a scheduled snack or meal
- sickness (especially with vomiting or diarrhea)
- exercising more than usual.
Unless corrected, hypoglycemia will lead to unconsciousness, convulsions (seizures), and possibly death. Early symptoms of hypoglycemia include: anxious feeling, behavior change similar to being drunk, blurred vision, cold sweats, confusion, cool pale skin, difficulty in concentrating, drowsiness, excessive hunger, fast heartbeat, headache, nausea, nervousness, nightmares, restless sleep, shakiness, slurred speech, and unusual tiredness or weakness.
Symptoms of hypoglycemia can differ from person to person. It is important that you learn your own signs of low blood sugar so that you can treat it quickly. It is a good idea also to check your blood sugar to confirm that it is low.
You should know what to do if symptoms of low blood sugar occur. Eating or drinking something containing sugar when symptoms of low blood sugar first appear will usually prevent them from getting worse, and will probably make the use of glucagon unnecessary. Good sources of sugar include glucose tablets or gel, corn syrup, honey, sugar cubes or table sugar (dissolved in water), fruit juice, or non-diet soft drinks. If a meal is not scheduled soon (1 hour or less), you should also eat a light snack, such as crackers and cheese or half a sandwich or drink a glass of milk to keep your blood sugar from going down again.
You should not eat hard candy or mints because the sugar will not get into your blood stream quickly enough. You also should not eat foods high in fat such as chocolate because the fat slows down the sugar entering the blood stream. After 10 to 20 minutes, check your blood sugar again to make sure it is not still too low.
Tell someone to take you to your doctor or to a hospital right away if the symptoms do not improve after eating or drinking a sweet food. Do not try to drive, use machines, or do anything dangerous until you have eaten a sweet food.
If severe symptoms such as convulsions (seizures) or unconsciousness occur, the patient with diabetes should not be given anything to eat or drink. There is a chance that he or she could choke from not swallowing correctly. Glucagon should be administered and the patient’s doctor should be called at once.
When to use glucagon injection
Glucagon is for use when someone is suffering severe hypoglycemia and is unable to treat themselves.
Glucagon may be given if the patient is:
- unconscious
- having a seizure
- unable to take anything sweet to raise their blood glucose
- or the patient has taken glucose by mouth which has not produced a raise in their blood glucose levels
Glucagon dosage
The dose of glucagon will be different for different patients. Follow your doctor’s orders or the directions on the label. The following information includes only the average doses of glucagon. If your dose is different, do not change it unless your doctor tells you to do so.
The amount of glucagon that you take depends on the strength of glucagon. Also, the number of glucagon doses you take each day, the time allowed between doses, and the length of time you take the medicine depend on the medical problem for which you are using glucagon injection.
For glucagon injection dosage form:
- As an emergency treatment for severe hypoglycemia:
- Adults and children 6 years and older and weighing 25 kilograms (kg) or more: 1 milliliter (mL) injected under your skin, into a muscle, or into a vein. The dose may be repeated while waiting for emergency assistance.
- Children younger than 6 years of age and weighing less than 25 kg: 0.5 mL injected under your skin, into a muscle, or into a vein.
If it becomes necessary to inject glucagon, a family member or friend should know the following:
- After the glucagon injection, turn the patient on his or her left side. Glucagon may cause some patients to vomit and this position will reduce the possibility of choking.
- The patient should become conscious in less than 15 minutes after glucagon is injected, but if not, a second dose may be given. Get the patient to a doctor or to hospital emergency care as soon as possible because being unconscious too long can be harmful.
- When the patient is conscious and can swallow, give him or her some form of sugar. Glucagon is not effective for much longer than 1½ hours and is used only until the patient is able to swallow. Fruit juice, corn syrup, honey, and sugar cubes or table sugar (dissolved in water) all work quickly. Then, if a snack or meal is not scheduled for an hour or more, the patient should also eat some crackers and cheese or half a sandwich, or drink a glass of milk. This will prevent hypoglycemia from occurring again before the next meal or snack.
- The patient or caregiver should continue to monitor the patient’s blood sugar. For about 3 to 4 hours after the patient regains consciousness, the blood sugar should be checked every hour.
- If nausea and vomiting prevent the patient from swallowing some form of sugar for an hour after glucagon is given, medical help should be obtained.
Keep your doctor informed of any hypoglycemic episodes or use of glucagon even if the symptoms are successfully controlled and there seem to be no continuing problems. Complete information is necessary for the doctor to provide the best possible treatment of any condition.
Replace your supply of glucagon as soon as possible, in case another hypoglycemic episode occurs.
You should wear a medical identification (ID) bracelet or chain at all times. In addition, you should carry an ID card that lists your medical condition and medicines.
Glucagon side effects
Glucagon may cause side effects. Tell your doctor if any of these symptoms are severe or do not go away:
- nausea
- vomiting
- rash
- itching
If you experience any of the following symptoms, call your doctor immediately:
- difficulty breathing
- loss of consciousness
Rare side effects:
- anxiety
- blurred vision
- chills
- cold sweats
- coma
- confusion
- cool, pale skin
- depression
- dizziness
- fast heartbeat
- headache
- increased hunger
- nausea
- nervousness
- nightmares
- seizures
- shakiness
- slurred speech
- unusual tiredness or weakness
Incidence not known:
- difficulty with swallowing
- dizziness, faintness, or lightheadedness when getting up suddenly from a lying or sitting position
- fast, pounding, or irregular heartbeat or pulse
- hives, itching, or skin rash
- pounding in the ears
- puffiness or swelling of the eyelids or around the eyes, face, lips, or tongue
- slow or fast heartbeat
- sweating
- tightness in the chest
Other side effects not listed may also occur in some patients. If you notice any other effects, check with your healthcare professional.
References- Glucagon and regulation of glucose metabolism. Am J Physiol Endocrinol Metab 284: E671–E678, 2003. https://www.physiology.org/doi/pdf/10.1152/ajpendo.00492.2002
- Wewer Albrechtsen NJ, Kuhre RE, Pedersen J, Knop FK, Holst JJ. The biology of glucagon and the consequences of hyperglucagonemia. Biomarkers Med 2016;10:1141–1151
- Gosmanov NR, Gosmanov AR, Gerich JE. Glucagon Physiology. [Updated 2011 Feb 4]. In: De Groot LJ, Chrousos G, Dungan K, et al., editors. Endotext [Internet]. South Dartmouth (MA): MDText.com, Inc.; 2000-. Available from: https://www.ncbi.nlm.nih.gov/books/NBK279127
- Tasaka Y, Inoue S, Hirata Y, Hanyu F, Endo M: Time course of content of insulin, glucagon and pancreatic polypeptide in human pancreatic tissue obtained by surgery. Endocrinol Jpn 28:261-264, 1981
- Gerich JE, Charles MA, Grodsky GM: Characterization of the effects of arginine and glucose on glucagon and insulin release from the perfused rat pancreas. J Clin Invest 54:833-841, 1974
- Galbo H, Holst JJ, Christensen NJ: Glucagon and plasma catecholamine responses to graded and prolonged exercise in man. J Appl Physiol 38:70-76, 1975
- Wilmore DW, Lindsey CA, Moyland JA, Faloona GR, Pruitt BA, Unger RH: Hyperglucagonaemia after burns. Lancet 1:73-75, 1974
- Rocha DM, Santeusanio F, Faloona GR, Unger RH: Abnormal pancreatic alpha-cell function in bacterial infections. N Engl J Med 288:700-703, 1973
- Gerich J, Davis J, Lorenzi M, Rizza R, Bohannon N, Karam J, Lewis S, Kaplan R, Schultz T, Cryer P: Hormonal mechanisms of recovery from insulin-induced hypoglycemia in man. Am J Physiol 236:E380-385, 1979
- Rizza RA, Cryer PE, Gerich JE: Role of glucagon, catecholamines, and growth hormone in human glucose counterregulation. Effects of somatostatin and combined alpha- and beta-adrenergic blockade on plasma glucose recovery and glucose flux rates after insulin-induced hypoglycemia. J Clin Invest 64:62-71, 1979
- Feldman JM, Plonk JW, Bivens CH: The role of cortisol and growth hormone in the counter-regulation of insulin-induced hypoglycemia. Horm Metab Res 7:378-381, 1975
- Lecavalier L, Bolli G, Cryer P, Gerich J: Contributions of gluconeogenesis and glycogenolysis during glucose counterregulation in normal humans. Am J Physiol 256:E844-851, 1989
- Jelinek LJ, Lok S, Rosenberg GB, Smith RA, Grant FJ, Biggs S, Bensch PA, Kuijper JL, Sheppard PO, and Sprecher CA. Expression cloning and signaling properties of the rat glucagon receptor. Science 259: 1614–1616, 1993.
- Burcelin R, Katz EB, and Charron MJ. Molecular and cellular aspects of the glucagon receptor: role in diabetes and metabolism. Diabetes Metab 22: 373–396, 1996.
- Role of fructose 2,6-bisphosphate in the control of glycolysis in mammalian tissues. Biochem J. 1987 Jul 15; 245(2): 313–324. https://www.ncbi.nlm.nih.gov/pmc/articles/PMC1148124/pdf/biochemj00251-0010.pdf
- The role of fructose 2,6-bisphosphate in the regulation of carbohydrate metabolism. Curr Top Cell Regul. 1984;23:57-86. https://www.ncbi.nlm.nih.gov/pubmed/6327193
- Hormonal control of pyruvate kinase activity and of gluconeogenesis in isolated hepatocytes. Proc Natl Acad Sci U S A. 1976 Aug; 73(8): 2762–2766. https://www.ncbi.nlm.nih.gov/pmc/articles/PMC430732/pdf/pnas00038-0236.pdf
- Quesada I, Todorova MG, Soria B. Different metabolic responses in alpha-, beta-, and delta-cells of the islet of Langerhans monitored by redox confocal microscopy. Biophys. J. 2006;90:2641–2650.
- Mitrakou A, Ryan C, Veneman T, Mokan M, Jenssen T, Kiss I, Durrant J, Cryer P, Gerich J: Hierarchy of glycemic thresholds for counterregulatory hormone secretion, symptoms, and cerebral dysfunction. Am J Physiol 260:E67-74, 1991
- Gromada J, et al. Adrenaline stimulates glucagon secretion in pancreatic A-cells by increasing the Ca2+ current and the number of granules close to the L-type Ca2+ channels. J. Gen. Physiol. 1997;110:217–228.
- MacDonald PE, et al. A K ATP channel-dependent pathway within alpha cells regulates glucagon release from both rodent and human islets of Langerhans. PLoS Biol. 2007;5:e143
- Franklin I, Gromada J, Gjinovci A, Theander S, Wollheim CB. Beta-cell secretory products activate alpha-cell ATP-dependent potassium channels to inhibit glucagon release. Diabetes. 2005;54:1808–1815
- Ravier MA, Rutter GA. Glucose or insulin, but not zinc ions, inhibit glucagon secretion from mouse pancreatic alpha-cells. Diabetes. 2005;54:1789–1797
- Cooperberg BA, Cryer PE. Beta-cell-mediated signaling predominates over direct alpha-cell signaling in the regulation of glucagon secretion in humans. Diabetes Care. 2009;32:2275–2280
- Dunning BE, Foley JE, Ahrén B. Alpha cell function in health and disease: influence of glucagon-like peptide-1. Diabetologia. 2005;48:1700–1713
- Meier JJ, Kjems LL, veldhuis JD, Lefèbvre P, Butler PC. Postprandial suppression of glucagon secretion depends on intact pulsatile insulin secretion: further evidence for the intraislet insulin hypothesis. Diabetes. 2006;55:1051–1056
- Bollheimer LC, et al. Stimulatory short-term effects of free fatty acids on glucagon secretion at low to normal glucose concentrations. Metabolism. 2004;53:1443–1448
- Ahrén B. Autonomic regulation of islet hormone secretion—implications for health and disease. Diabetologia. 2000;43:393–410
- Dumonteil E, et al. Glucose regulates proinsulin and prosomatostatin but not proglucagon messenger ribonucleic acid levels in rat pancreatic islets. Endocrinology. 2000;141:174–180
- Postprandial Suppression of Glucagon Secretion: A Puzzlement. Diabetes May 2017, 66 (5) 1123-1125; DOI: 10.2337/dbi16-0075 http://diabetes.diabetesjournals.org/content/66/5/1123
- De Oya M, Prigge WF, Swenson DE, Grande F. Role of glucagon on fatty liver production in birds. Am. J. Physiol. 1971;221:25–30
- Caren R, Corbo L. Transfer of plasma lipid to platelets by action of glucagon. Metabolism. 1970;19:598–607.
- Caren R, Corbo L. Glucagon and plasma arachidonic acid. Metabolism. 1965;14:684–692.
- Amatuzio DS, Grande F, Wada S. Effect of glucagon on the serum lipids in essential hyperlipemia and in hypercholesterolemia. Metabolism. 1962;11:1240–1249
- De Oya M, Prigge WF, Grande F. Suppression by hepatectomy of glucagon-induced hypertriglyceridemia in geese. Proc. Soc. Exp. Biol. Med. 1971;136:107–110.
- Eaton RP. Hypolipemic action of glucagon in experimental endogenous lipemia in the rat. J. Lipid Res. 1973;14:312–318
- Guettet C, Mathé D, Riottot M, Lutton C. Effects of chronic glucagon administration on cholesterol and bile acid metabolism. Biochim. Biophys. Acta. 1988;963:215–223
- Guettet C, et al. Effect of chronic glucagon administration on lipoprotein composition in normally fed, fasted and cholesterol-fed rats. Lipids. 1991;26:451–458
- Guettet C, Mathé D, Navarro N, Lecuyer B. Effects of chronic glucagon administration on rat lipoprotein composition. Biochim. Biophys. Acta. 1989;1005:233–238
- Guettet C, Rostaqui N, Navarro N, Lecuyer B, Mathe D. Effect of chronic glucagon administration on the metabolism of triacylglycerol-rich lipoproteins in rats fed a high sucrose diet. J. Nutr. 1991;121:24–30.
- Rudling M, Angelin B. Stimulation of rat hepatic low density lipoprotein receptors by glucagon. Evidence of a novel regulatory mechanism in vivo. J. Clin. Invest. 1993;91:2796–2805
- Bobe G, Ametaj BN, Young J. w., Beitz DC. Effects of exogenous glucagon on lipids in lipoproteins and liver of lactating dairy cows. J. Dairy Sci. 2003;86:2895–2903.
- Bobe G, Sonon RN, Ametaj BN, Young JW, Beitz DC. Metabolic responses of lactating dairy cows to single and multiple subcutaneous injections of glucagon. J. Dairy Sci. 2003;86:2072–2081
- Conarello SL, et al. Glucagon receptor knockout mice are resistant to diet-induced obesity and streptozotocin-mediated beta cell loss and hyperglycaemia. Diabetologia. 2007;50:142–150.
- Longuet C, et al. The glucagon receptor is required for the adaptive metabolic response to fasting. Cell Metab. 2008;8:359–371.
- Richter w. O., Robl H, Schwandt P. Human glucagon and vasoactive intestinal polypeptide (VIP) stimulate free fatty acid release from human adipose tissue in vitro. Peptides. 1989;10:333–335.
- Lefebvre P, Luyckx A, Bacq ZM. Effects of denervation on the metabolism and the response to glucagon of white adipose tissue of rats. Horm. Metab. Res. 1973;5:245–250.
- Perea A, Clemente F, Martinell J, villanueva-Peñacarrillo ML, valverde I. Physiological effect of glucagon in human isolated adipocytes. Horm. Metab. Res. 1995;27:372–375.
- Nair KS, welle SL, Halliday D, Campbell RG. Effect of beta-hydroxybutyrate on whole-body leucine kinetics and fractional mixed skeletal muscle protein synthesis in humans. J. Clin. Invest. 1988;82:198–205
- Gerich JE, et al. Prevention of human diabetic ketoacidosis by somatostatin. Evidence for an essential role of glucagon. N. Engl. J. Med. 1975;292:985–989
- Pegorier JP, et al. Induction of ketogenesis and fatty acid oxidation by glucagon and cyclic AMP in cultured hepatocytes from rabbit fetuses. Evidence for a decreased sensitivity of carnitine palmitoyltransferase I to malonyl-CoA inhibition after glucagon or cyclic AMP treatment. Biochem. J. 1989;264:93–100
- vons C, et al. Regulation of fatty-acid metabolism by pancreatic hormones in cultured human hepatocytes. Hepatology. 1991;13:1126–1130.
- Charbonneau A, Couturier K, Gauthier MS, Lavoie JM. Evidence of hepatic glucagon resistance associated with hepatic steatosis: reversal effect of training. Int. J. Sports Med. 2005;26:432–441.
- Charbonneau A, Unson CG, Lavoie JM. High-fat diet-induced hepatic steatosis reduces glucagon receptor content in rat hepatocytes: potential interaction with acute exercise. J. Physiol. 2007;579(Pt 1):255–267.
- Charbonneau A, Melancon A, Lavoie C, Lavoie JM. Alterations in hepatic glucagon receptor density and in Gsalpha and Gialpha2 protein content with diet-induced hepatic steatosis: effects of acute exercise. Am. J. Physiol. Endocrinol. Metab. 2005;289:E8–E14
- Eaton RP, Schade DS. Glucagon resistance as a hormonal basis for endogenous hyperlipaemia. Lancet. 1973;1:973–974
- Song KH, Chiang JY. Glucagon and cAMP inhibit cholesterol 7alpha-hydroxylase (CYP7A1) gene expression in human hepatocytes: discordant regulation of bile acid synthesis and gluconeogenesis. Hepatology. 2006;43:117–125
- Chiang JY. Bile acid regulation of gene expression: roles of nuclear hormone receptors. Endocr. Rev. 2002;23:443–463.
- Hylemon PB, et al. Hormonal regulation of cholesterol 7 alpha-hydroxylase mRNA levels and transcriptional activity in primary rat hepatocyte cultures. J. Biol. Chem. 1992;267:16866–16871.
- Davidson IW, Salter JM, Best CH. The effect of glucagon on the metabolic rate of rats. Am. J. Clin. Nutr. 1960;8:540–546.
- Nair KS. Hyperglucagonemia increases resting metabolic rate in man during insulin deficiency. J. Clin. Endocrinol. Metab. 1987;64:896–901.
- Calles-Escandón J. Insulin dissociates hepatic glucose cycling and glucagon-induced thermogenesis in man. Metabolism. 1994;43:1000–1005.
- Kuroshima A, Yahata T. Thermogenic responses of brown adipocytes to noradrenaline and glucagon in heat-acclimated and cold-acclimated rats. Jpn. J. Physiol. 1979;29:683–690.
- Yahata T, Habara Y, Kuroshima A. Effects of glucagon and noradrenaline on the blood flow through brown adipose tissue in temperature-acclimated rats. Jpn. J. Physiol. 1983;33:367–376.
- Billington CJ, Briggs JE, Link JG, Levine AS. Glucagon in physiological concentrations stimulates brown fat thermogenesis in vivo. Am. J. Physiol. 1991;261(Pt 2):R501–R507.
- Edwards CI, Howland RJ. Adaptive changes in insulin and glucagon secretion during cold acclimation in the rat. Am. J. Physiol. 1986;250(Pt 1):E669–E676
- Billington CJ, Bartness TJ, Briggs J, Levine AS, Morley JE. Glucagon stimulation of brown adipose tissue growth and thermogenesis. Am. J. Physiol. 1987;252(Pt 2):R160–R165.
- Cypess AM, et al. Identification and importance of brown adipose tissue in adult humans. N. Engl. J. Med. 2009;360:1509–1517
- Dicker A, Zhao J, Cannon B, Nedergaard J. Apparent thermogenic effect of injected glucagon is not due to a direct effect on brown fat cells. Am. J. Physiol. 1998;275(Pt 2):R1674–R1682
- Filali-Zegzouti Y, et al. Role of catecholamines in glucagon-induced thermogenesis. J. Neural Transm. 2005;112:481–489.
- Morales A, et al. Sympathetic control of glucagon receptor mRNA levels in brown adipose tissue of cold-exposed rats. Mol. Cell Biochem. 2000;208:139–142
- Brito NA, Brito MN, Bartness TJ. Differential sympathetic drive to adipose tissues after food deprivation, cold exposure or glucoprivation. Am. J. Physiol. Regul. Integr. Comp. Physiol. 2008;294:R1445–R1452.
- Watanabe J, Kanai K, Kanamura S: Glucagon receptors in endothelial and Kupffer cells of mouse liver. J Histochem Cytochem 36:1081-1089, 1988
- Heppner KM, Habegger KM, Day J, Pfluger PT, Perez-Tilve D, Ward B, Gelfanov V, Woods SC, DiMarchi R, Tschop M: Glucagon regulation of energy metabolism. Physiol Behav 100:545-548, 2010
- Geary N, Smith GP: Selective hepatic vagotomy blocks pancreatic glucagon’s satiety effect. Physiol Behav 31:391-394, 1983
- Kurose Y, Kamisoyama H, Honda K, Azuma Y, Sugahara K, Hasegawa S, Kobayashi S: Effects of central administration of glucagon on feed intake and endocrine responses in sheep. Anim Sci J 80:686-690, 2009
- Penick SB, Hinkle LE., Jr. Depression of food intake induced in healthy subjects by glucagon. N. Engl. J. Med. 1961;264:893–897.
- Martin JR, Novin D. Decreased feeding in rats following hepatic-portal infusion of glucagon. Physiol. Behav. 1977;19:461–466
- Le Sauter J, Geary N. Hepatic portal glucagon infusion decreases spontaneous meal size in rats. Am. J. Physiol. 1991;261(Pt 2):R154–R161
- Langhans W, Pantel K, Müller-Schell W, Eggenberger E, Scharrer E. Hepatic handling of pancreatic glucagon and glucose during meals in rats. Am. J. Physiol. 1984;247(Pt 2):R827–R832.
- Le Sauter J, Noh U, Geary N. Hepatic portal infusion of glucagon antibodies increases spontaneous meal size in rats. Am. J. Physiol. 1991;261(Pt 2):R162–R165.
- Geary N, Kissileff HR, Pi-Sunyer FX, Hinton v. Individual, but not simultaneous, glucagon and cholecystokinin infusions inhibit feeding in men. Am. J. Physiol. 1992;262(Pt 2):R975–R980
- Geary N, Le Sauter J, Noh U. Glucagon acts in the liver to control spontaneous meal size in rats. Am. J. Physiol. 1993;264(Pt 2):R116–R122
- Martin JR, Novin D, vanderweele DA. Loss of glucagon suppression of feeding after vagotomy in rats. Am. J. Physiol. 1978;234:E314–E318.
- Geary N, Smith GP. Selective hepatic vagotomy blocks pancreatic glucagon’s satiety effect. Physiol. Behav. 1983;31:391–394.
- Kurose Y, et al. Effects of central administration of glucagon on feed intake and endocrine responses in sheep. Anim. Sci. J. 2009;80:686–690.
- Gelling R. w., et al. Lower blood glucose, hyperglucagonemia, and pancreatic alpha cell hyperplasia in glucagon receptor knockout mice. Proc. Natl Acad. Sci. USA. 2003;100:1438–1443